August 1965 Electronics World
Table
of Contents
Wax nostalgic about and learn from the history of early electronics. See articles
from
Electronics World, published May 1959
- December 1971. All copyrights hereby acknowledged.
|
Side-looking airborne radar started out using a narrow beam formed
by reflectors, like traditional radars, as opposed to the synthetic
aperture type most often (maybe even exclusively) used today. Both
types of side-looking radars rely primarily on the physical movement
of the airborne platform for effective azimuthal scanning rather
than steering the beam either mechanically or electronically. Modern
computer-controlled synthetic radar beams can be segmented and directed
off-axis for detected areas of interest as required, but the early
systems simply gathered radar return data and presented it real-time,
with some level of analog processing, to operators.
Side-Looking Radar Imagery
By J. L. Nelson
Military Electronics Division Motorola Inc.
Side-looking radars mounted in our military reconnaissance planes
provide near-photographic mapping coverage in clouds and darkness.
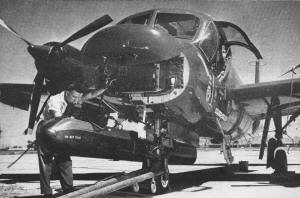
Fig. 1. - Side-looking radar installation on
an Army Mohawk aircraft. Notice the long cigar-shaped radar antenna
housing mounted beneath the fuselage.
Imagery produced by electronic sensors, such as radar and infrared,
is playing an increasingly greater role in the reconnaissance efforts
of the military services. Such imagery not only supplements the
aerial photograph, but in certain instances provides information
that cannot be obtained by photographic techniques. For example,
it has long been established that the ability of various types of
electronic sensors to penetrate clouds and darkness as well as to
map large areas is a most desirable characteristic.
Side-Looking Radar
An example of an electronic sensor currently used for mapping
purposes is the AN/UPD-2 Side-Looking Radar System produced by Motorola's
Military Electronics Division for the U. S. Army. The name "side-looking"
stems from the fact that this form of radar collects mapping information
from the terrain to the sides of the aircraft. In contrast, infrared
and photographic systems are generally used to record terrain beneath
the aircraft. Radar as a Sensor is a ranging device; its maximum
range limit is approximately equal to the line-of-sight distance
to the horizon.
For example, an aircraft flying at 3,000 feet above the terrain
is capable of mapping in excess of 50 miles to each side of the
aircraft during a single run. It is not uncommon to map areas in
excess of 30,000 square miles during the course of a single run,
and this record may be contained on a strip of film less than 2
feet long. To better understand the structure of a radar image,
the mechanics of the technique must be examined.
Fig. 1 illustrates a typical side-looking radar installation
in the Army's Mohawk aircraft. The cigar-shaped structure beneath
the aircraft is the radar antenna.
Fig. 2 shows the geometry peculiar to a side-looking radar. Note
that the antenna pattern is a narrow fan-shaped beam extending from
the aircraft outward to the horizon. In general, this beam is less
than 10° thick; therefore, the radar illuminates and receives
returns from only a narrow strip of terrain at anyone time. As the
aircraft moves forward, successive strips of terrain are viewed
by the radar system.
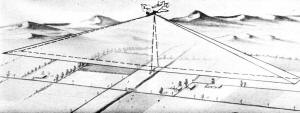
Fig. 2. - Geometry of a side-looking radar that
has been installed on a reconnaissance aircraft.
As contrasted to a camera, a radar system does not examine the
entire area contained in the strip at one time. It accomplishes
its purpose by transmitting a high-energy pulse and recording the
intensity of the return echo in synchronism with the time required
for the pulse to reach a particular element of terrain and return
to the aircraft.
Thus, the basic terrain information is contained in terms of
"range vs. time" as a video signal. This signal is converted to
a film record by placing the video information on a cathode-ray
tube as intensity modulation, sweeping the cathode-ray tube in synchronism
with the radar return from each element of terrain, and photographing
the resultant display. The film is caused to move at a rate proportional
to the ground speed of the aircraft, thereby building up the map
in synchronism with the illumination of successive strips of ground
by the radar antenna. In general, the ground-scanning process may
be likened to the scanning spot of a television raster.
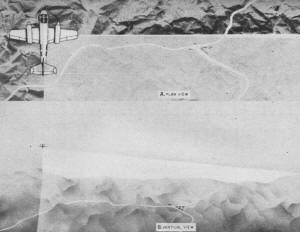
Fig. 3. - Coverage patterns obtained with side-looking
radar. (A) Pattern as viewed from above. (B) View from in front
of plane.
The process, as viewed from a point directly above the surveillance
aircraft, is illustrated in Fig. 3. The narrow light area perpendicular
to the side of the aircraft represents the geometry of the radar
beam; hence the strip of terrain under surveillance. The area immediately
aft of this beam represents terrain already mapped by the sensor.
Examples of Imagery
A typical example of imagery produced by a side-looking radar is
shown in Fig. 4. The horizontal dimension is related to radar slant
range. The black central stripe represents the range to the ground
directly beneath the aircraft. Features such as roads, cities, farms,
and other landmarks are readily discernible. Another example of
a radar map made in the area of Phoenix, Arizona is shown in Fig.
5.
Imagery such as that of Figs. 4 and 5 does not always lend itself
to direct correlation with terrain maps or other systems of coordinates
because of various types of distortion. Two prominent forms of imagery
distortion often found in radar strip maps are: drift-angle distortion
and ground-speed distortion.
Taking first the case of an aircraft encountering a side wind,
it will be necessary for the pilot to intentionally "crab" or yaw
the aircraft to maintain the desired ground track. If the antenna
is rigidly affixed to the aircraft for aerodynamic reasons, the
beam will no longer be perpendicular to the ground track but will
be rotated by an angle equal to the drift angle of the aircraft.
In general, side-looking radar systems compensate for this by rotating
the intensity-modulated line scan on the cathode-ray tube a proportionate
amount, thereby providing first-order correction.
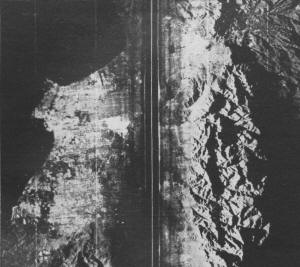
Fig. 4. Side-looking radar imagery of Los Angeles
area. The black central stripe which resembles a roadway directly
below the plane is the aircraft's line of flight. Distortion Effects
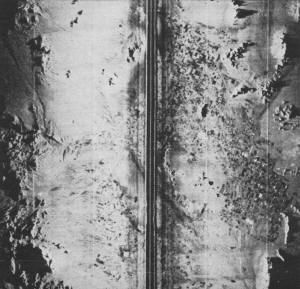
Fig. 5. Side-look radar imagery of Phoenix, Arizona
area. Notice the outward-going shadows on both sides of the center
stripe resulting from radar beam illumination.
To avoid ground-speed distortion, it is necessary to synchronize
the motion of the film across the image plane to that of the aircraft
over the terrain. In the event such synchronization is not achieved,
the scale factor lengthwise along the film record will not be in
agreement with the scale factor laterally across the film record.
Thus, it is necessary that two vital pieces of information be provided
to render proper coordinates on the final imagery.
One device used for obtaining this basic information is a Doppler
navigator.
However, present Doppler navigators provide drift-angle and ground-speed
information to accuracies on the order of 1 to 2 percent. After
this information is further processed by the radar system, this
error may be increased by another 1 or 2 percent.
A number of higher order distortions are often apparent in a
radar photograph. Such second-order effects as slant-range distortion
and calibration accuracy and third-order effects such as cathode-ray
tube and lens pin-cushion or barrel distortion, film shrinkage,
and other factors may be present. Although a trained interpreter
with suitable viewing equipment can often compensate for such distortions,
the radar system design should minimize them as far as practical.
For practical, day-to-day utility, a mapping radar requires other
characteristics often overlooked by systems designers. Foremost
among these is stability-freedom from drift of important parameters
such as video gain, CRT intensity and focus, etc. Since the map
is recorded photographically, it is not feasible for an operator
to "tweak" controls to obtain optimum imagery.
The future of radar holds much promise in improving radar surveillance
imagery. Fig. 4 demonstrates the dramatic improvements in map realism
now consistently obtainable through better scanning geometry, higher
resolution, and longer grey scale. Further improvements in resolution
could result from the use of shorter wavelengths and/or longer antennas.
The first is negated by rain and fog attenuation and the second
by aerodynamic considerations.
The effects of long antennas can be synthesized by complex signal
processing. Although such techniques hold the promise of future
resolution improvements, equipment based on these principles is
not yet dependable enough for field use by military personnel.
Posted May 11, 2015
|