November 1970 Popular Electronics
Table of Contents
Wax nostalgic about and learn from the history of early electronics. See articles
from
Popular Electronics,
published October 1954 - April 1985. All copyrights are hereby acknowledged.
|
"One of the least known but
most fascinating of semiconductor devices is the light-emitting diode (LED). Until
quite recently, these devices were too expensive for widespread use." That statement
is hard to imagine in 2023. It was written 50 years ago by Mr. Forest Mims, in
this November 1970 issue of Popular Electronics magazine. Now, LEDs
seem to be in every consumer device, whether it be a simple power ON indicator
light or an array of alpha-numeric displays. TV and stereo remotes use infrared
LEDs, opt isolators that use LEDs are integral components of modems, motor
controllers, and motion sensors. Commercial truck and some passenger vehicle
tail lights are made of LED clusters, as are
the very expensive LED bulbs that are quickly replacing incandescent and,
thankfully, the
toxic (mercury) CFL household bulbs. What was once rare is now a commodity - it
happens all the time.
Light Emitting Diodes - New Semiconductors for Readout and Communication
Cover story by Forrest M. Mims, III
One of the least known but most fascinating of semiconductor devices is the light-emitting
diode (LED). Until quite recently, these devices were too expensive for widespread
use; however, technological advances in their fabrication now make possible moderately
low prices so that they are attractive to the electronics experimenter.
The first recorded instance of light being generated by a "diode" was in 1907
when H. J. Round touched a pair of battery wires to a crystal of silicon carbide.
Much to his surprise, flashes of yellow light were emitted at the contact region
of one of the battery wires - he had accidentally discovered the LED. Unfortunately,
his discovery was forgotten and not until the early 1950's did scientists once again
study semiconductor light emission. At that time several patents were applied for
covering LED's made from silicon or germanium - common semiconductor materials.
One of these patents not only described the principle of the LED but listed several
fascinating uses for the device. Among them were light-beam communication systems,
light "radar", and light beam alignment devices.
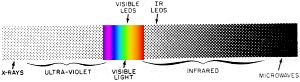
Fig. 1 - Most infrared LED's emit light at a wavelength
of about 0.9 microns of the electromagnetic spectrum. Visible LED's may emit from
0.53 microns to the limit of visible region at about 0.75 microns.
Unlike the 1907 silicon carbide cat whisker diode, the 1950's LED's emitted infrared
light (see Fig. 1). The invisible beam was desirable but researchers began
a concentrated effort to fabricate LED's in the visible range. The first company
to market any kind of LED was Texas Instruments, Inc. Their diodes included infrared
emitters made from gallium arsenide (GaAs) and visible emitters made of gallium
arsenide phosphide (GaAsP). These diodes were expensive; but their appearance on
the commercial market in 1962 whetted the appetites of design engineers - and of
course was of great interest to other semiconductor manufacturers. While scientists
at IBM and Bell Telephone performed basic research on the devices (especially visible
emitters), General Electric, Monsanto, Electro-Nuclear Laboratories and others began
competing with TI.
Since the LED has an almost unlimited lifetime and because of its low
operating current, many claims were made for its potential in flat screen
television, indicator lamps, night lights, and even as a source of room
lighting. The extremely fast modulation capability of the LED made possible
several demonstrations of voice communications permitting two parties to
converse over a beam of invisible infrared light for
clear weather distances of several miles.
How Does It Do It?
The LED is different from conventional incandescent
lamps because the latter give off light as a byproduct of heat. That is, the filament
must first be heated to incandescence before light is produced. This, of course,
is the reason for placing incandescent filaments in evacuated or gas-filled glass
bulbs. If the filament were exposed to oxygen, it would quickly be consumed. Not
so with LED's; they operate with or without the presence of air. In fact, the main
reason LED's are placed in containers is to protect the rather delicate contact
wires. Often, in fact, the LED is simply protected by a layer of clear epoxy which
serves both as a protective cover and a lens. Since the LED produces far more light
than heat, it is a more efficient source of light than the incandescent lamp.
Production of light by an LED comes under the broad heading of "electro-luminescence."
Luminescence describes light produced by means other than incandescence. Since luminescing
bodies are generally at the temperature of their environment, they are sometimes
referred to as sources of "cold light." Fireflies, many species of fish, rotting
wood, marsh gas, and many other objects are sources of luminescent light.
Light from an LED is the result of stimulation by a small electric current. As
is the case with all luminescent bodies, the light is a result of sub-atomic events.
The cause of these events may be chemical, as with the firefly, or electrical as
with the LED.
Light Generation
In the LED, emission of light is called "PN
junction luminescence." As shown in Fig. 2, light is emitted at the junction
of an LED when electrons which have been stimulated to higher than normal energy
levels move across the junction and fall back into their normal places. The energy
loss resulting when an electron reoccupies its normal slot in an atom is accompanied
by the emission of a photon of light at a wavelength related to the difference in
energy between the two bands. Since junction luminescence involves the combination
of electrons with holes, physicists often refer to the effect as "recombination
radiation."

Fig. 2 - Light is emitted when an electron crosses the np
junction interface and falls from the excited to the unexcited state.

Fig. 3 - The critical interface problem. Much more light
is emitted by hemispheric diode but some is lost in the thick n-region. Some planar
emitters are provided with a reflector to give greater light output.
The sub-atomic physics of semiconductor light emission can be highly efficient.
But the mechanisms of bringing the light to the surface and the application of electrical
current to the diode can be terribly inefficient. Sources of inefficiency are:
1. Internal absorption of light within the p or n region of the semiconductor
following its emission at the junction.
2. Reabsorption of light which is reflected back into the diode due to the "critical
interface" problem.
3. Resistance at the electrical contacts and in the semiconductor.
Many of these inefficiencies have been partially resolved as a result of years
of research. Consider, for example, the critical interface problem. Since most semiconductors
have a high index of refraction, they tend to reflect light at air-semiconductor
interfaces. The effect is similar to that of an observer looking through a glass
window and seeing his own reflection as well as objects on the other side of the
window. The effect results in the loss of light that is generated at the junction.
An ingenious solution to the problem was suggested by early research and was
first used commercially by Texas Instruments. It consists of forming the light emitting
region of the diode into a dome. Since the light is only bent and not reflected
back into the diode, all light reaching the surface is emitted (see Fig. 3).
Unfortunately, the dome approach does have its drawbacks - the longer path that
the light must travel before being emitted causes more internal absorption than
in planar emitters. Also, grinding domed diodes is an expensive process.
In an effort to gain the benefits of both planar and domed diodes, many manufacturers
now coat planar diodes with a transparent dome of epoxy which essentially serves
the same purpose of the semiconductor dome.
Physics of Light Emitting Diodes
Modern atomic physics tells us that electrons within the confines of a particular
atom are allowed to occupy only discrete energy levels or bands. Energy levels between
the outer two bands, the valence and conduction bands, are separated by a region
called the forbidden gap. Under special conditions of doping, an electron may occupy
one or more levels within the forbidden gap for relatively brief periods of time.
The forbidden gap plays an important role in semiconductor light emission, since
transitions from the conduction to the valence hand provide the mechanism for photon
generation.
An efficient way to cause transitions between the valence and conduction bands
is to pump or inject electrons into the n region of a semiconductor diode. If a
sufficient number of electrons is injected, the potential barrier at the junction
of the n and p regions will be overcome and current will flow through the diode.
Having crossed the junction barrier, the injected electrons seek their equilibrium
point and drop from their excited position in the conduction band to the valence
band. In essence, the electrons drop into holes or regions in a band where there
is an electron deficiency.
The act of electrons combining with holes is called recombination and results
in the magic of PN junction electroluminescence or light emission. An electron falling
from a high to a low level releases the energy which propelled it over the junction
as heat, light, or some of each. Heat emission is accompanied by vibrations in the
crystal lattice and if left uncontrolled, results in thermal destruction of the
diode. Heat emission predominates in indirect band gap semiconductors such as silicon
and germanium. The forbidden gap in such materials permits an electron falling from
the conduction to the valence band to loiter briefly at one or more levels. Transitions
between the various levels in the forbidden gap may result in either light or heat
emission.
It is interesting to note that an ordinary silicon or germanium diode emits a
minute quantity of infrared light when forward biased. However, light emission in
such diodes is far less efficient than heat production and develop-ment of practical
semiconductor light emitters followed research with direct band-gap materials.
Gallium arsenide GaAs is normally a direct band-gap material. Since electrons
injected into a GaAs diode recombine with holes without pausing at intermediate
levels within the forbidden gap, light emission is very efficient and production
of heat is generally limited to contact resistance and bulk absorption of some of
the emitted light within the semiconductor.
GaAs diodes are so efficient as light emitters that scientists chose them as
candidates for early work concerning the feasibility of fabricating semiconductor
lasers. In the fall of 1962, researchers at G.E., IBM, and MIT announced almost
simultaneously lasers made from specially prepared GaAs diodes. In a future issue
of POPULAR ELECTRONICS, the operating principles and characteristics of the diode
laser will be described.
How Are They Made?
As mentioned earlier, the LED usually consists
of a PN junction of one type of semiconductor, the most common being gallium arsenide.
A typical diode may consist of a small wafer of positively charged (p) material
in intimate electrical contact with a metal header similar to a transistor case.
Before it is mounted on the header, the wafer is given a thin top layer of negatively
charged (n) GaAs by either diffusion or epitaxial growth. The P portion of the finished
wafer is connected to one of the wire leads of the header, resulting in a true PN
junction diode.
To collect the light output from the wafer in an efficient manner, a metal can
with a lens or flat window at one end is welded to the header. The can also serves
as protection (see Fig. 4).

Fig. 4 - In practical applications, the LED is mounted within
a metal enclosure, similar to a transistor, for mechanical protection. A transparent
window permits the light to exit from one end of the enclosure.
How Are They Used? Now that we know something about the history,
physics, and mechanical construction of the LED, what are its applications other
than those found in the laboratory? Especially, how can the experimenter use these
tiny sources of "cold light."
An important military use of the LED is as a covert source of illumination for
night vision devices. The usefulness of the Army's starlight scope is greatly enhanced
by invisible infrared illumination supplied by one or more LED's. Another military
use is in covert communications. The Navy, for example, has several types of infrared,
line-of-sight LED voice communicators for ship-to-ship and ship-to-shore.
Recently, commercial applications of the LED have been revealed at an unprecedented
rate. Several companies are mass producing tiny alpha-numeric displays consisting
of arrays of LED's. These displays operate at a much lower voltage than conventional
mechanical, incandescent, cathode ray, or gas-discharge displays.
One manufacturer has demonstrated a complete VOM in a probe! Using several of
the new numeric displays, the futuristic unit is similar in size to a standard scope
probe. Volts and ohms are read directly from the small light display. The Hamilton
Watch Company will market a digital watch using LED numeric displays, and Bell Telephone
is hard at work on new types of blue, green, yellow, and red LED's for use in home
telephones. Infrared LED's are being used in several types of new intrusion alarms.
One company even markets a complete line of LED voice communicators and burglar
alarms.
One of the most unusual applications of the infrared LED is as a light source
for small mobility aids for the blind. Such devices may eventually be marketed at
a price comparable to most hearing aids.
An important result of all these new uses for LED's is a large drop in price.
LED's are available for under $2.50 each in small quantities. This price is competitive
with that of miniature, long-life indicator lamps. And of course, the LED offers
sturdier packaging, a million-hour lifetime, and far less current consumption.
Only a year ago, the cheapest infrared LED retailed for $18.00. At least five
manufacturers now offer GaAs LED's for under $7.50. These devices are far more efficient
than visible LED's and are therefore usable in experiments in secret communications.
To sum up then, the LED is superior to the conventional filament lamp in the
following ways:
1. Response time is extremely fast - most LED's operate in a fraction of a second
rise and fall time . Some LED' can reach a speed of 100 MHz.
2. Because it is a solid-state device, an LED has no warm-up time, is completely
free of microphonics, is just about impervious to mechanical vibration and other
environmental conditions, and is usually of very small size and weight.
3. The LED light output is nearly monochromatic-far from being a laser - but
close enough to have most of its light in a relatively narrow bandwidth. This makes
it possible to use optical filters to reduce ambient noise.
4. The LED is a low-impedance device with forward characteristics similar to
those of a conventional silicon diode and it can be driven from ordinary low-voltage
supplies with conventional transistor circuitry.
Elsewhere in this issue you will find a unique construction article for a low-cost
LED communicator. Other projects using LED's will appear in future issues.
More Information
For more information about commercial light emitting diodes, write to one of
the following manufacturers:
- Electro·Nuclear Laboratories 115 Independence Drive Menlo Park, CA 94025
- Miniature Lamp Department General Electric Company Nela Park Cleveland, OH 44112
- Monsanto Electronic Special Products 10131 Bubb Road Cupertino, CA 95014
- Radio Corporation of America Electronic Components and Devices Harrison, NJ
07029
- Texas Instruments Semiconductor Components Division Box 5012 Dallas, TX 75222
- Fairchild Semiconductor 313 Fairchild Drive Mountain View, CA 94040
- Hewlett-Packard 1501 Page Mill Road Palo Alto, CA 94304
- Motorola Semiconductor Products Box 20912 Phoenix, AZ 85036
- Sharp Electronics Corporation 178 Commerce Road Carlstadt, NJ 07072
- An excellent booklet on LED's is sold by the General Electric Company for $2.00.
Called the "Solid State Lamp Manual," it describes in detail theory, characteristics,
and applications.
Posted April 19, 2023 (updated from original post
on 9/16/2011)
|