October 1949 Popular Science
[Table of Contents]
Wax nostalgic about and learn from the history of early
electronics. See articles from
Popular
Science, published 1872-2021. All copyrights hereby acknowledged.
|
Here is a really good
synopsis of "rare earth" elements that explains how they came to be known in
that way. Hint: It is not that they are so rare, in fact per Wikipedia,
Cerium is the
25th most abundant element on Earth. The issue is they are not in concentrated
lodes, but spread out as components of other mineral compounds, so extensive
processing is needed to isolate and purify them. One of the first post-war
commercial level extraction processes was the result of experimentations during
nuclear bomb research. As you might know, "holes" existed in the Periodic Table
of the Elements when it was first constructed in 1869 by
Dmitri
Mendeleev, because not all predicted naturally occurring elements had been
found. Helium, atomic
number 2, was not found on Earth until 1895, after first having been observed in
the sun's spectrum a few years earlier (hence its name, from Helios). Author
Alden Armagnac provides a primer in the original 15 rare earths (now 17) in this
1949 Popular Science magazine article.
Rare Earth Metals, Once Forgotten, Now in Production
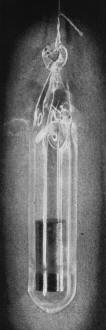
Metals of atomic age may have names like praseodymium, thanks
to new process for purifying rare earths.
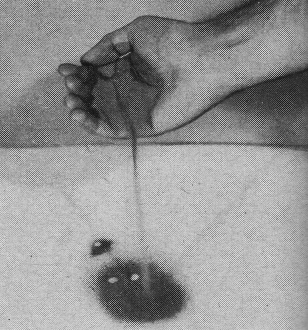
Worth 31 times its weight in gold, silvery praseodymium
metal in vial at left is sealed from air, which turns it black. One source of rare-earth
metals is monazite sand, above.
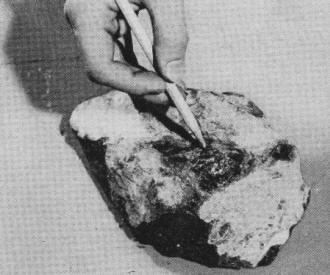
Glossy black portion of gadolinite ore, pointed
out above, also yields many rare-earth elements. Ore and one of metals, gadolinium,
are named after Finnish rare-earth chemist Gadolin.
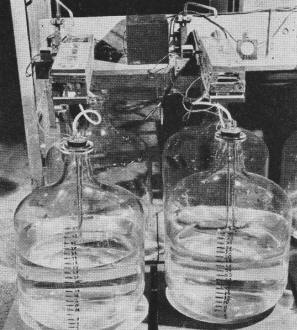
Ingenious control automatically switches output
of column from full to empty collecting bottle. Rising liquid trips electric circuit,
shifting carriage holding funnels and delivery tubes.
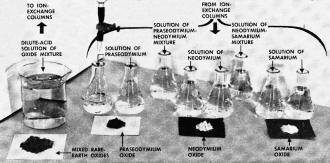
Pictorial diagram shows separation process at
a glance. Rare-earth oxide mixture, dissolved in acid (in beaker) goes into columns.
Rinsing with citric acid and ammonium citrate yields series of "fractions" in flasks,
of which last obtained is beneath delivery tube. From pure fractions, oxalic acid
precipitates oxalates, converted by heat to pure oxides shown.
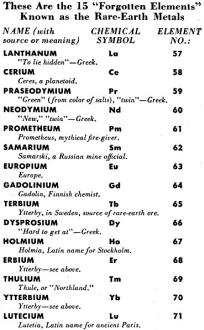
These Are the 15 "Forgotten Elements" Known as the Rare-Earth
Metals
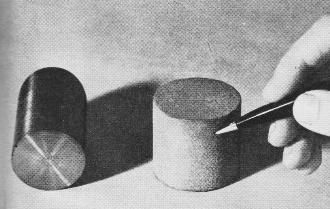
Air gives cerium, right, this cream-colored
coating. Freshly machined lanthanum, left, shines like steel. Each sample of rarely
seen metal weighs 1.3 lb. and is valued at $1,200.
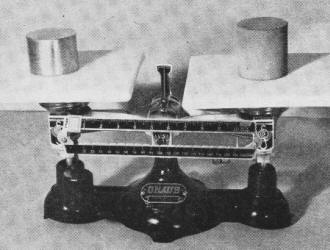
Rare-earth metals are only moderately heavy.
Cerium on right-hand side of scales, with density 6.8 times that of water, is balanced
by smaller piece of copper with density of 8.9.
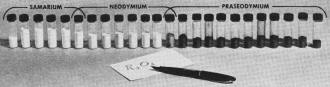
Yields of rare earths in oxide form, from series
of 12-hour runs through columns, show almost total separation of test mixture of
equal parts of samarium, neodymium, and praseodymium.

Results of another test show successful recovery
of neodymium and praseodymium from complicated mixture of other rare earths including
hard-to-separate gadolinium and samarium.
By Alden P. Armagnac
Spang in the middle of Iowa there's something nearly as tall as the corn - and
more exciting. It's a bank of glass tubes. Out of them come strange metals, some
of the most precious on earth, whose unusual properties may prove vital to the atomic
age.
They once were rarer than diamonds and actually priceless. Even now, being produced
in pound lots by a method born of the atom-bomb project, they cost up to 31 times
their weight in gold.
Few people have ever seen them. Gray to light gray with high metallic luster
at first, they turn odd shades on exposure to air - pale yellow, cream color, black,
grayish pink. Their compounds vie in brilliant hues with the colors of the rainbow.
Called the "rare-earth" metals, they are true chemical elements, just as iron,
copper, and silver are. Their names sound like something out of an apothecary shop
- lanthanum, cerium, praseodymium, neodymium, to mention a few.
Small wonder if the names are new to you. For the whole group of 15 rare-earth
metals, listed on page 143, might well be called the "forgotten elements." They
make up nearly one-sixth of the 96 known elements, the basic materials of which
all things are made. Yet a typical college textbook on chemistry, more than 1,000
pages long, devotes a scant two pages to them.
Practical uses have been as few in proportion. Just one may be familiar to most
laymen. Spin the wheel of your cigarette lighter, and you are striking fire from
pyrophoric, or spark-producing, rare earths, cerium metal in particular, in the
alloy of the flint. Lanthanum oxide goes into special optical glass for aerial-camera
lenses. Neodymium oxide absorbs glare in glass-blowers' goggles. Carbon-arc projectors
in movie theaters give an intense white light because of rare earths in the carbons.
There have been a few other uses for rare earths, but not many.
Today, however, these forgotten elements have become news. Atomic research has
focused the spotlight upon them. And under the direction of Dr. Frank H. Spedding,
Iowa State College chemist, the Ames Laboratory of the U. S. Atomic Energy Commission
has begun producing highly purified rare-earth metals and compounds in unprecedented
quantity.
One object is to see if they may be useful materials for future atomic power
plants - for instance, in control rods and in lightweight shielding against dangerous
radiation from atomic engines of ships and planes. Forming as "ashes" in uranium
rods of piles, rare earths have been a nuisance, tending to put out the atomic fires
by absorbing neutrons. But that very vice would be a virtue in shielding and control.
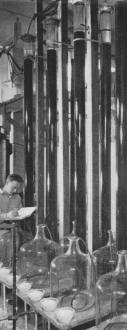
Dozens of these eight-foot-tall ion-exchange
columns, at Ames, Iowa, "refinery," accomplish feat of separating rare-earth elements,
which are almost identical chemically.
"Moreover," says the U. S. Atomic Energy Commission, "it now appears that they
may have a bright commercial future as alloy metals in the manufacture of high-temperature
structural materials."
It's partly the small proportion of rare earths in the earth's crust - there's
as much gold in sea water - that makes them "rare," not scarcity of places where
they can be found. One widely distributed ore is the yellow-to-brown monazite sand
of the Carolinas, Brazil, Africa, and India. Norwegian mines furnish gadolinite,
a glossy black rare-earth ore, and others. Rare earths of commerce have been relatively
inexpensive "concentrates" or crude mixtures obtained from these ores. For example,
cerium for cigarette-lighter flints is a $4-a-pound commercial alloy known as "mischmetal,"
consisting of cerium and seven other rare-earth metals, and other impurities.
But one of the biggest reasons the rare earths are so "rare" is their unusual
chemical resemblance to each other. They are so much alike that ordinary chemical
methods cannot separate one from the others. Years of tedious evaporating and re-dissolving
were formerly necessary to get a few specks of material.
Then in early 1944, workers on the atom-bomb project, including those at Ames,
Iowa, made the amazing discovery that rare earths formed by uranium fission could
be separated - in hours instead of years - simply by passing a solution of them
through a vertical tubeful of synthetic resin. Samples were, at first, sub-visible
"tracer" quantities; later, visible ones of ten-thousandths of an ounce.
Exciting news came from Clinton Laboratories at Oak Ridge, Tenn., when two young
chemists separated a mysterious substance from fission-produced neodymium with one
of the new resin tubes or "ion-exchange columns." It proved to be the long-missing
rare-earth element No. 61, which they named prometheum. Radio-active and short-lived,
it is believed non-existent in nature. First visible samples, pink and yellow smears
on white porcelain disks, are minute quantities of prometheum nitrate and chloride
respectively.
In the meantime, Dr. Spedding of Iowa State proposed as bold a step-up in scale
as that from a pinhead of plutonium to pounds of it for the bomb. Instead of being
content with "micro" quantities of pure rare earths, why not adapt the new method
to produce them in "macro" amounts of grams or even pounds? He and his colleagues
installed a battery of 24 resin columns, eight feet tall and four inches in diameter
- and made history by accomplishing the feat.
Watching the precious liquids trickle from glistening glass columns into collecting
bottles the size of cider jugs, a visitor to this pilot plant hardly realizes the
miracle taking place in the tubes.
Into the top of a tube goes a hodgepodge of rare-earth elements - re-dissolved
in acid after acid extraction from the ore and crude preliminary chemical separation
into two main groups, light and heavy.
Like flies to flypaper, ions - individual charged atoms - of rare earths stick
to the resin at the upper end of the tube. Thus they are strained-"adsorbed" is
the technical word - from the acid, which is then removed by washing.
Down Go the Ions
Now a dilute solution of citric acid and ammonium citrate is poured down the
column. Rinsed from the resin, or "eluted" if you prefer the scientific term, a
typical rare-earth ion starts down the tube. Almost at once it sticks to the resin
again. This skip-stop progress repeats itself, over and over, all the way to the
tube's bottom.
Eventually all the rare-earth particles reach bottom. But those of the element
of highest atomic number get there first; those of the next highest number, next,
and so on. Switch the liquid output from one collecting bottle to another, at the
right time intervals, and nearly every bottleful or "fraction" will contain a single
purified rare-earth element. A few fractions in which elements overlap are re-treated;
and purified fractions, if still higher purity is wanted, may also be run through
a column again.
The solutions yield oxalates, by precipitation with oxalic acid. Then heat treatment
converts these to the rare-earth oxides - the form desired for many experiments
and from which other compounds may readily be prepared. Purity runs around 99.9
per-cent.
By "smelting" rare-earth oxides when enough are on hand, the Ames workers produce
miniature ingots of the pure metals-tiny 1/20-oz. cylinders of silvery praseodymium
and neodymium metals, sealed against corroding air in helium-filled glass vials,
and comparatively massive 1.3-lb. chunks of cerium and lanthanum metals. Their weight
compares with iron, their softness with calcium; like the latter, they react with
water, liberating hydrogen. Producing one avoirdupois ounce of praseodymium costs
almost $1,000; of neodymium, $170; of lanthanum and cerium, $57 each - compared
with the $31.90 value of an ounce of pure gold ($35 per troy ounce).
If new uses require pure metals, even these prices - which the experimenters
hope to reduce - may not be commercially prohibitive. Like radium in watch dials,
a little may go a long way. Or like platinum in chemical crucibles, advantages may
outweigh cost. But it is primarily for research that scientists prize the newly
available pure preparations. At last their long-mysterious properties can be fully
explored for possible exploitation. The "forgotten elements" are forgotten no longer.
Posted April 16, 2024
|