May 1963 Radio-Electronics
[Table of Contents]
Wax nostalgic about and learn from the history of early electronics.
See articles from Radio-Electronics,
published 1930-1988. All copyrights hereby acknowledged.
|
A while back, I posted the "Battery
Types and Their Characteristics" article from the April 1973 issue of Popular
Electronics magazine. While preparing it I ran across Eric Wrobble's
vintage battery collection.
All of the battery types shown in the lead photo of this 1963 Radio-Electronics
"Inside the Dry Cell" article can be found amongst them. Back in the day, energy
storage density was low compared to the chemistries employed in modern cells. Nickel
cadmium (NiCad) dominated the rechargeable battery market for dry cell types. Lead-acid
cells did and still do pack a lot of energy into a given volume of space, but they
are very heavy and can be potentially destructive if the electrolyte finds it way
outside the shell. In the early days of radio controlled model airplanes, wet cells
were often used for powering the airborne electronics, and many otherwise survivable
crashes resulted in loss of the model because acid leaked from a crack in the battery
pack. Today, lithium polymer (LiPo) and lithium ion (Li-Ion) cells and battery packs
rule the portable electrical device world. They pack an enormous amount of energy
into a volume, recharge quickly, and are very safe... at least until a breach in
the containment wrapper permits humid air or water to come into contact with the
lithium, or improper charging causes thermal runaway and the whole thing bursts
into flames. There were very few -if any - reports of any of the dry cell varieties
shown here catching fire.
Inside the Dry Cell
By Gordon E. Kaye*
Dry cells can look alike but be very different inside. Left to right, size-D
cells for: radio use, flashlights, photography, heavy-duty service, transistor radios,
all-purpose (manganese) and a mercury transistor battery. In the center, of course,
the familiar little hearing-aid or miniature-radio mercury cell.
What is the difference between a zinc-carbon and an alkaline cell? How do they
both differ from a mercury cell? And what does it mean to the service technician
or battery user?
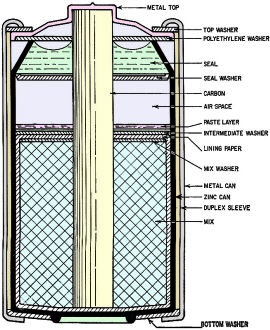
Fig. 1 - Cross-section of typical size-D zinc-carbon cell.
Universal battery exists for every application in today's electronics. The user
must consider what he needs from a battery system. Then he can choose the battery
on the basis of electrical characteristics and physical dimensions.
First, we have to ask: What is a dry cell? We hear such titles as "energizer"
and "activator", and even "alkaline energizer", and we all know that there is a
mercury battery. How do these cells differ, and why?
Dry primary (nonrechargeable) cells and batteries convert chemical energy into
electrical energy, on periodic or continuous demand, over a long storage period.
They are "dry" only because their liquid acid or alkali electrolyte is held in a
leak-resistant container.
All commercially available dry cells produce their electrical energy by oxidizing
or corroding an active metal, such as zinc, in an electrolytic solution. Zinc is
the negative terminal and is called the anode. The oxygen which corrodes it comes
from an oxide of mercury (HgO) or of manganese (MnO2). These oxides form
the positive terminal or cathode. (Note well that the carbon "positive pole" of
a dry cell takes no part in the reaction - it is simply a convenient conductor.)
The cathode is also called the depolarizer since its oxygen depolarizes or reacts
with the hydrogen formed at the anode. This removes a cause of high internal resistance
at this terminal.
We will stay with the three types of commercially available primary dry cells
generally found in electronic devices. These are the Leclanché, or zinc-carbon,
the manganese-alkaline-zinc and the mercury cell.
Zinc-carbon (Leclanché) cell
The Leclanché cell provides an average of 20 watt-hours per pound in most
applications. It is inefficient at heavy loads and will probably provide about 10
watt-hours per pound in heavy-duty service. At very light loads, 25 watt-hours per
pound is available.
A typical cell is shown in Fig. 1. Chemically this cell consists of manganese
dioxide (MnO2) as the active cathode and depolarizer, sal ammoniac (ammonium
chloride) as an electrolyte, with additions of zinc chloride and mercuric chloride
in water. These are blended with graphite or carbon black in a mix. This carbon
black actually takes no part in the reaction, but is used to improve current-carrying
capacity in the partially conductive MnO2 depolarizer, and to serve as
an absorbent for the electrolyte. The inert, porous carbon rod down the center acts
both as a positive terminal and a gas vent. An extruded zinc container serves as
an anode.
The relatively impure, low-cost MnO2 is a cause of poor shelf life.
The impurities in this natural ore cause side reactions which in time degrade the
cell.
A very pure synthetic MnO2 has been developed to provide a more efficient
oxygen-bearing material. When blended with natural ore, this electro-ore can improve
battery performance.
The Leclanché cell requires constant venting to relieve internal pressure.
However, venting lets water vapor escape from the cell at higher temperatures. This
in turn raises the internal resistance by drying out the electrolyte. The overall
low shelf life of the zinc-carbon system is partly due to these slow internal losses,
and in smaller cells the problems become aggravated.
The MnO2 depolarizer gives up only 30-50% of its available oxygen.
It is rapidly converted to Mn2O3 a compound much less conductive
than the original. This accounts for the sharp drop in voltage with discharge time.
The Leclanché cell suffers from oxygen starvation during the latter part of its
life. Since there is some remaining zinc in the can, the chemical reaction continues
and hydrogen accumulates when the load is left on the cell. The pressure developed
by the hydrogen distorts the can or bursts it so its corrosive contents spill out.
Steel-jacketed cells are preferable in preventing equipment damage.
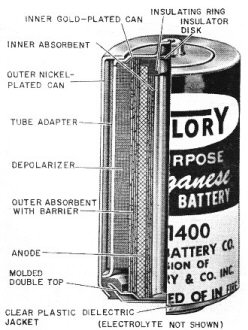
Fig. 2 - A manganese-alkaline-zinc cell. Electrolyte is not shown.
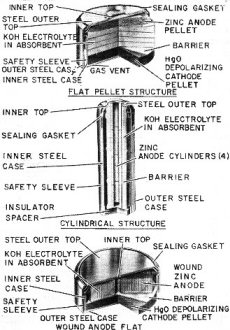
Fig. 3 - Cutaway views of cylindrical, flat-pellet and wound-anode
mercury cells.
The Leclanché cell, under heavy loads, should be used intermittently.
Poor depolarizer efficiency allows hydrogen gas to accumulate. It appears as an
internal resistance in series with the load, so it must be given time to dissipate.
(No extra energy is gained during the rest period. You can't get more out of a battery
than was built into it.
Because of the wide range of applications and drain rates, four grades of cells
are supplied. They are listed in the table. Most battery manufacturers make the
four types and list applications on the label.
While the carbon content is greater in the photoflash mix, the cell capacity
is reduced accordingly. Flash bulbs need heavy current but little total energy per
flash. The opposite is true of radios, where drains are light and many hours are
needed. Capacity is increased by using electro-ore in the mix.
The zinc-carbon cell is the work-horse of the battery industry. It combines low
initial cost with availability in many sizes and shapes. It has been much improved
in efficiency and design since it first appeared in 1868.
Manganese-alkaline-zinc cell
This system was developed jointly by Ruben Laboratories and P. R. Mallory &
Co. The cell in Fig. 2 will deliver 30 to 40 watt-hours per pound at good rates
with high overall efficiency, The depolarizer or cathode is electro-ore with a small
percentage of graphite. Density is made high by forming the oxide pellets under
many tons of pressure, improving conductivity and making a maximum of oxygen available.
Due partly to the large amount of de-polarizer, the alkaline cell does not leak
or burst if left in a discharged state. The alkali electrolyte is 40% potassium
hydroxide (KOH) solution, presaturated with zinc so the zinc anode will not dissolve
during normal shelf stand or storage.
The negative zinc anode is pressed from a highly purified zinc powder amalgamated
with pure mercury to minimize adverse local action. The anode is placed inside the
cell and is not used as a container. Some cells are built with anodes in a gel structure
holding the electrolyte and a dispersed zinc powder. This increases anode surface
area and results in high conductivity.
Mercuric-alkaline-zinc cell
This cell, shown in its three structural types in Fig. 3, is considered the best
commercially available dry cell today. It was developed in 1942 by Dr. Samuel Ruben.
It packs 45 watt-hours per pound and 6 watt-hours per cubic inch-with high efficiency
and excellent voltage regulation.
The mercury cell doesn't need a rest between loads because of the ease with which
available oxygen is released from the mercuric oxide. This takes place in a simple
conversion with 100% efficiency. Another factor is the low internal resistance of
these cells, even in small sizes. Interrupted or continuous loads have little effect
on the total energy delivered.
Composition of Leclancé Cells
Electrochemistry of a typical mercury cell is illustrated in Fig. 4. If no resistor
or other load were in the circuit, the no-load voltage of the cell would be the
net sum of the electro-chemical reactions of zinc and mercuric oxide with respect
to the alkaline electrolyte. The zinc, dissolving or ionizing in the electrolyte,
leaves electrons behind and leaves the zinc anode with a negative charge. Likewise,
positive charges accumulate at the mercuric oxide cathode.
With the load resistor across the cell, the cell voltage forces the accumulated
electrons at the anode to flow in the direction shown. Reducing the anode charge
causes the electrochemical balance to be upset, and the zinc again starts going
into solution as zinc ions (Zn++): At the cathode, the mercuric oxide
combines with water to form Hg(OH)2. This separates into mercury ions
(Hg++) and two hydroxyl ions, 2(OH)-. The hydroxyl ions react
with the zinc to form zinc oxide (a blue-white paste) and water (H2O).
There is no net loss of water in this cell system.
When the electrons pass through the resistor,
they give up their energy and end up at the cathode. The mercury ions (Hg++)
grab two electrons each and become free mercury. This is seen in a disassembled
expended cell as globules of quicksilver in an inert graphite slurry.
The above reactions take place through a permeable barrier (usually a special
high-grade paper or porous plastic material. The barrier passes ions but prevents
solid particles from migrating and causing internal short circuits.
The inert, plated steel container is one of the reasons for the high reliability
of the mercury system. It is not eroded away or weakened by chemical action, so
it does not swell or leak if left on load after complete discharge. These features
appear also in Mn-alkaline systems. Including excess depolarizer prevents hydrogen
formation at the end of life.
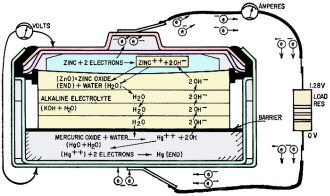
Fig. 4 - Electrochemical action in typical mercury cell.
The shelf life of the mercury battery is excellent. Shelf stands of 8 years at
room temperature showed 65 % to 70% remaining capacity. Cells 12 to 13 years old
showed 25% to 40% remaining at room temperature. In both the mercury and manganese-alkaline
systems, the high-temperature storage problem is improved. At a continuous 120°
F, these batteries show about 25% of their room-temperature capacity in a year,
without serious leakage. Good-quality mercury or manganese cells are suitable where
3 to 5 years delayed service in normal environments is expected.
Another article will compare the three common types in ordinary applications,
showing which battery should be used for given jobs and the reasons for the choice.
* Applications engineer, Mallory Battery Co.
Posted May 12, 2023
|