By now, engineers and scientists
have managed to replace most vacuum tubes with solid state devices - at least for
consumer products. The one place tubes remain are in microwave oven. Those klystron
tubes operate in the 2.4 GHz band and typically output ranging from about 500 W
to 2 kW. No doubt materials and methods have changed since the 1950s, but fundamentally
klystrons of today are the same as klystrons then. Between this article and
Part 1
that appeared in the April 1952 issue of Radio & Television News magazine,
authors Joseph Racker and Lawrence Perenic provide a very nice introduction on the
topic. According to the Wikipedia entry, the name "klystron" comes from the Greek
verb κλύζω (klyzo) referring to the action of waves breaking against a shore, and
the suffix -τρον ("tron") meaning the place where the action happens. The name "klystron"
was suggested by Hermann Fränkel, a professor in the classics department at Stanford
University when the klystron was under development.
Microwave Klystron Oscillators
By Joseph Racker* and Lawrence Perenic†
Part 2. Concluding article of this series covering the use of reflex klystrons
in modern circuitry.
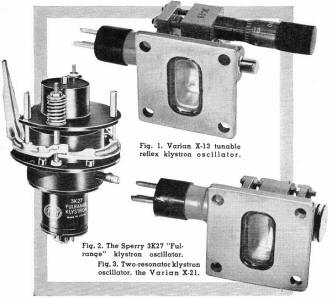
Fig. 1 - Varian X-13 tunable reflex klystron oscillator.
Fig. 2 - The Sperry 3K27 "Full-range" klystron oscillator.
Fig. 3 - Two-resonator klystron oscillator, the Varian X-21.
While the two-resonator klystron, described last month, represents the simplest
type for purposes of discussion and explanation, it is far from the simplest type
to operate.
For instance, it was seen in Part 1 how the alternating voltage across the catcher
had to be timed to retard the bunches of electrons as they arrived. Since the rate
at which these bunches arrived was governed by the frequency of the alternating
voltage across the buncher, it was evident that both the buncher resonant circuit
and the catcher resonant circuit have to be tuned to the same frequency for proper
operation. Now, inasmuch as the resonant circuits associated with all klystrons
are actually high-"Q" cavities, it becomes difficult to keep two or more such circuits
in tune, thus tuning of a two-resonator klystron represents a serious problem.
This problem of tuning is responsible, to a large extent, for the fact that of
all forms of klystrons, by far the most common is the reflex klystron which utilizes
only one resonant cavity. In the reflex klystron the same cavity is used for both
bunching and catching. Instead of traveling only once through a straight drift-space,
as in the two-resonator klystron, the beam is turned back on itself by a retarding
field. This field is produced by a reflector plate which is maintained at a negative
potential with, respect to the cathode, as shown in Fig. 4. The retarding field
is strong enough not only to prevent the electrons from arriving at the reflector,
but also to return them to the buncher.
Upon examining Fig. 4 it can be seen that the method of producing velocity-modulation
in a reflex klystron is basically the same as that used in a two-resonator klystron.
An electron which passes the center of the buncher at the instant the alternating
voltage across the grids is passing through zero has the same net voltage acting
on it as it had when it passed through the accelerator grid. It, therefore, leaves
the buncher with the same velocity it had when it entered. An electron which passes
through the center of the buncher a little later, however, when the alternating
voltage is increasing in the positive direction, has a higher net voltage acting
on it. As a result, this electron leaves the buncher with a higher velocity than
it had when it entered. It is seen, therefore, that in a manner similar to the one
which took place in the two-resonator klystron, electrons passing through the buncher
grids are either accelerated, decelerated, or remain unaffected by the buncher field,
depending upon the phase and amplitude of the buncher voltage at the time they pass
through.
Reflector Bunching Action
As was stated previously, the retarding field of the reflector is made sufficiently
strong not only to prevent the electrons from arriving at the reflector, but also
to return them to the buncher. In this way it becomes feasible to use only one pair
of grids and one resonant cavity to act as both buncher and catcher. Since there
is no longer a field-free drift space in which the velocity-modulated electrons
can become bunched, "reflector-bunching" is the mechanism by which density-modulation
is achieved in the reflex klystron, and the operation is as follows:
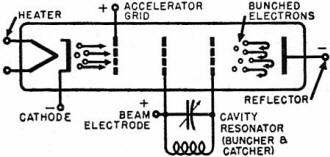
Fig. 4 - Schematic diagram of reflex klystron.
Conditions for Oscillation
In general, the condition for optimum operation of a reflex klystron is the same
as for the two-resonator klystron, namely, the center of the bunches in the stream
should pass through the catcher when the catcher field has its greatest retarding
effect. Under this condition the greatest number of electrons lose the largest possible
amount of power during the transit and the maximum power is extracted from the beam.
In the case of the reflex klystron, which has no separate catcher, this means that
the electron stream should be returned to the buncher when the buncher field has
its maximum retarding effect.
It can be shown that this condition is satisfied if the total drift time of an
electron, i.e., the time it takes to travel from the buncher to the region of the
reflector and return to the buncher, equals 1 3/4 cycles. That is, any change in
the total reflector transit time from this value of 1 3/4 cycles results in a decrease
in power delivered to the resonant circuit. If the total transit time is increased
to 2 3/4 cycles, however, optimum conditions will exist once more because the phase
of the catcher field will again be such as to retard the returning electrons. Similarly,
for other values such as:
(n + 3/4) cycles
where n is a whole number such as 1, 2, 3, etc., oscillations may exist.
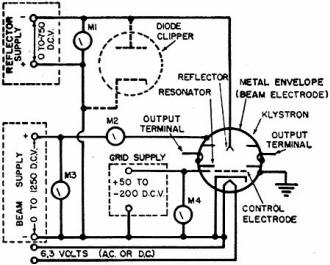
Fig. 5 - Correct power supply connections for a reflex klystron
oscillator.
This does not mean to imply that the reflex klystron will always oscillate if
this transit time condition is met. If the d.c. beam current is too small, the power
delivered by the bunched beam to the catcher may be less than the power dissipated
in the buncher circuit. In this case there will be no oscillation, Therefore, a
second condition is also necessary for oscillation to be maintained, namely, the
d.c. beam current must exceed some minimum current, called the "starting current,"
which depends upon circuit and external load conditions. This starting current is
inversely proportional to (n + 3/4), that is, it is greater for transit times of
13/4 cycles than it would be for, say, 3 3/4 cycles. If both the conditions just
discussed, i.e., total transit time equal to (n + 3/4) cycles, and minimum starting
current are satisfied, oscillations will always occur.
Methods of Tuning
In actual practice there are several ways in which the transit time of a reflex
klystron may be adjusted to satisfy the first condition mentioned.
Fig. 5 shows, schematically, how such a klystron might be connected to power
supplies in a typical oscillator circuit. Four separate supplies are indicated;
a heater supply, a grid-bias supply, a beam supply, and a reflector supply. Of these,
the latter two can be adjusted (either singly, or in combination) to vary the transit
time and thus provide optimum operation. Most frequently, however, a change in reflector
voltage rather than a change in beam voltage is used to control the transit time
because a change in beam voltage produces two effects that tend to compensate each
other. Increasing the beam voltage results in a higher velocity for the electrons,
which tends to increase the time it takes them to return to the catcher; while at
the same time it produces a greater potential difference between the cavity and
the reflector, which tends to return the electrons in a shorter time. Since a change
in reflector voltage produces only the second of these effects, about one-third
as much change is needed in the reflector voltage as in the beam voltage for equal
results. In addition, the reflector is a high-impedance electrode which draws no
current and, therefore, the controlling elements (usually potentiometers) need not
be capable of handling any appreciable amounts of power.
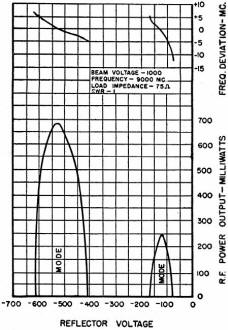
Fig. 6 - Characteristics of reflex klystron.
Since changing the transit time in a klystron is equivalent to varying the operating
frequency, it is seen that by adjusting the reflector or the beam voltage of these
tubes, the frequency may be changed. This behavior is known as "electronic tuning,"
and is utilized in practice to change the oscillating frequency by a comparatively
small percentage. The main frequency controlling element in the reflex klystron
is the resonant cavity which forms part of the buncher-catcher. By mechanically
compressing the cavity, the volume of the cavity and the spacing of the grids connected
to it are caused to change and, in this way, alter the oscillating frequency. This
is called "mechanical tuning." In practice, both of the above methods are used,
as follows:
The mechanical tuning is adjusted so that the operating point falls in a region
of operation where the frequency can be shifted a reasonable amount in either direction
with electronic tuning without too much change in output power. The beam voltage
and grid bias are adjusted to provide at least minimum starting current. Once the
tube is oscillating, the reflector voltage is adjusted to optimize the transit time
and thus provide maximum power output within a given "mode" of oscillation.
Typical Behavior Curves
Klystron oscillators, in general, are characterized by the existence of discrete
"modes of oscillation." (The word mode is used here to denote certain limited regions
of electrode voltage within which oscillation occurs. Within a given mode, the frequency
of oscillation changes continuously from one end of the mode to the other as the
electrode voltage is changed.) The following are typical curves which illustrate
this behavior:
Fig. 6 illustrates the so-called "reflector characteristics" of a reflex klystron,
and shows both the variation of power output and the variation of frequency with
reflector voltage. As can be seen from the lower curves, there are several discrete
ranges of reflector voltage at which the tube will oscillate and supply power to
the load. It is these ranges of operation which were previously defined as "modes."
Inspection of the lower curves further reveals that the r.f. power outputs are not
the same for all modes. In general, the maximum power output is greater at the higher
reflector voltage modes. However, it is important to keep in mind that while the
maximum power output obtainable may differ from one mode to the next, the frequency
at the point of maximum output in each mode is exactly the same - being the resonant
frequency of oscillation of the cavity.
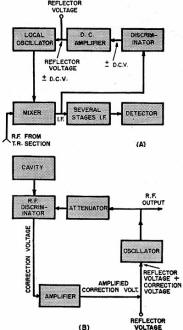
Fig. 7 - (A) The difference-frequency type of a.f.c. system.
(B) Block diagram of the absolute-frequency type of a.f.c. system.
In addition to showing the power output vs reflector voltage characteristics
just discussed, Fig. 6 also gives the frequency deviation vs reflector voltage characteristics
of a typical reflex klystron. These curves are drawn in the upper portion of the
graph and show the variation of output frequency with changes in the reflector voltage
within any one mode. As was previously defined, this shifting of the operating frequency
with reflector voltage is called "electronic tuning." The upper curves show that
the range of tuning also varies with the mode, but in the opposite sense; in general,
the range is smaller at the higher reflector voltage modes, making the operating
frequency less susceptible to change for a given ripple in the reflector voltage
supply. Since the modes with the highest output have the smallest electronic tuning
range, a compromise must often be made between the value of reflector voltage which
will yield the highest power output and a value of reflector voltage which will
result in the greatest tuning range.
Automatic Frequency Control
There are times when a very high degree of frequency stability is required and
then some form of a.f.c. is usually employed. Most a.f.c. systems operate to minimize
the difference between the frequency of the klystron oscillator and some standard
of reference. In most radar applications, the standard of reference is the transmitter
frequency plus or minus the intermediate frequency. This type of a.f.c. system can
be thought of as a difference-frequency system. Another type of a.f.c. system used
for the beacon local oscillator of an airborne radar uses a precision resonant cavity
as the standard of reference. This type of a.f.c. system can be thought of as an
absolute-frequency system.
An example of the difference-frequency type of system is shown in the block diagram
of Fig. 7A. The mixer, Lt. stages, and detector are similar to those found in any
radar receiver. During transmission a portion of the transmitted pulse leaks through
the "transmit-receive" section and is fed to the mixer in the same manner as would
be a received signal. The mixer, in turn, produces an i.f. output which is applied
to the discriminator of the a.f.c. system. The discriminator is able to distinguish.
between signals which are above the desired frequency and those which are below,
and is able to produce an output voltage which is positive in one case and negative
in the other. This d.c. voltage is amplified and added to the reflector voltage
of the local-oscillator klystron in such a way as to maintain the output of the
mixer at constant intermediate fre-quency.
In the absolute-frequency type of system, a high-"Q" reference cavity is employed
as the standard. A block diagram illustrating the basic circuit is shown in Fig.
7B. As can be seen from the diagram, a portion of the r.f. output from the klystron
oscillator is fed to an r.f. discriminator which is capable of producing an output
voltage in proportion to the difference between the oscillator frequency and the
resonant frequency of the cavity. This correction voltage is amplified and added
to the reflector voltage of the klystron in such a way as to make the oscillator
frequency the same as that of the reference cavity.
Details on Reflex Klystron
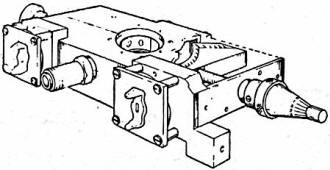
Fig. 8 - Typical cavity for 8·12 cm region.
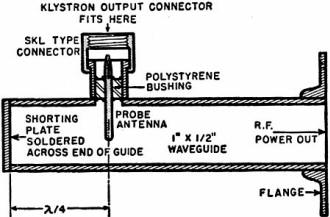
Fig. 9. Simple wave guide-to-coax transition.
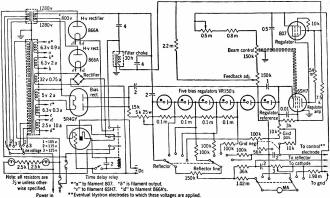
Fig. 10 - Schematic diagram of a satisfactory power supply for
reflex klystrons.

Fig. 11 - A 2K39 reflex klystron mounted on wave guide with which
it is used.

Fig. 12 - Simplified crystal detector circuit.
Although all reflex klystrons behave electronically in a similar manner to that
described in the foregoing paragraphs, in the past few years different mechanical
design approaches have resulted in the development of several distinctive types
of tubes. Since the most noticeable feature of a reflex klystron is its resonant
cavity, a convenient grouping is according to the location of the cavity; that is,
whether it is built into the tube (integral cavity), or external to it (external
cavity).
The 3K27 reflex klystron, for example, is an integral cavity type and is illustrated
in Fig. 2. The octal tube base serves to connect the proper operating voltages to
the heater, grid, and buncher. Part way up the tube, pointing to the right, is a
short length of rigid coaxial line which forms the output lead. The microwave oscillations
in the cavity are coupled out by means of an inductive pickup loop located inside
the cavity and sent through this coaxial line to the output connector where it is
available to do useful work. The cavity proper is the cylindrical portion of the
tube which starts just below the coaxial output line and extends upward as far .as
the helical springs.
The mechanical tuning mechanism, not shown in the photograph, consists of helical
springs, levers, tuning knob, screw, and a right-angle bracket. By rotating the
tuning knob, the tuning screw moves either in or out of the bracket. This causes
the levers to change position. Since the levers, in turn, are connected to the movable
wall of the cavity, the cavity shape becomes distorted and the frequency is changed
to suit the new cavity dimensions. The 3K27 reflex klystron, with a full-range tuner,
is capable of covering the entire frequency range of 770 to 1150 megacycles by rotation
of a single knob, and with an average power output of about 1 1/2 watts.
A second type of reflex klystron, known as the 2K28, uses an external cavity.
In this type of tube the resonant cavity does not lie completely within the tube,
but is chiefly external to it. In addition, the tube is constructed mainly of glass
and does not have any tuning mechanism built in. The tube base is located at the
bottom and serves to connect the proper operating voltages to the tube, as before.
The electron gun occupies the region just below a lower copper disc which protrudes
through the glass. This copper disc and the one above it form only a part of the
cavity. The remainder of the cavity, whatever type it may be, must make contact
with these two projecting discs. A typical cavity for the 8 to 12 cm region is shown
in Fig. 8. The 2K28 fits in the hole in the center of the cavity with its discs
making contact with the cavity walls.
Mechanical tuning is accomplished by turning the tuning knob, shown at the right-hand
side of Fig. 8, which causes the sliding plunger (shown in the cutaway section)
to move either toward or away from the klystron. This motion changes the volume
of the cavity and thus the frequency of operation. The microwave oscillations are
coupled out by means of an inductive pickup loop and fed through the short section
of coaxial line shown on the left-hand side of the cavity. The 2K28 reflex klystron
has a lead protruding through the top of the glass envelope which is used to connect
the reflector voltage to the tube. Although the 2K28 was designed to operate in
the 10 cm. range, oscillations have been obtained as low as 1000 mc. by using the
proper external cavity. The average power output of this tube is about 70 mw.
Having discussed the theoretical aspects of satisfactory klystron operation and
briefly described the physical details of some external and integral-cavity types,
this article concludes with some notes on the construction of an actual oscillator.
Fig. 11 shows a 2K39 reflex klystron, an integral-cavity type, mounted (within
a Plexiglas safety cover) directly on the wave guide with which it is to be used.
The tube is supported by means of a metal bracket to a wave guide-to-coax transition.
This transition, or adapter, consists of a section of wave guide 1" x 1/2" in cross-section,
shorted at one end, and with a probe projecting into the guide as shown in Fig.
9. The upper end of the probe ends in what is known as an "SKL" fitting, * which
mates with the "SKL" fitting on the output line of the klystron. Use of such a direct
mechanical coupling between the klystron and wave guide eliminates the annoying
tendency of the output power to vary - which occurs when non-rigid coaxial lines
are used at these frequencies. Moreover, the power output obtainable from the oscillator
is greater due to the decrease in length of the coupling line.
To operate the oscillator shown, two additional items are required: the first
is a power source capable of supplying the voltages previously discussed, the second
is a blower of approximately 50 cfm (cubic feet-per-minute) capacity to cool the
klystron at maximum ratings and to improve the frequency stability. Although several
excellent supplies are available commercially, the experimenter who wishes to construct
his own will have to exercise particular care with the reflector supply. The supply
should be designed to have a low impedance to reverse current to prevent a transient
condition or modulating voltage from driving the reflector positive - a condition
which can seriously damage the tube. A satisfactory supply should not have more
than a 50,000 ohm bleeder across its output. In addition, all three d.c. supplies
should be characterized by very low ripple content. The reflector supply variation,
in particular, must be held to better than a small fraction of one volt, while the
beam supply need be only one-half as good (on a percentage basis). Fig. 10 gives
the schematic of one of many supplies which has been found to give satisfactory
performance. Note in particular that the reflector supply is exceptionally well
regulated.
This oscillator may be tuned to any frequency in the 7500 to 10,300 megacycle
range with a power output of better than 300 mw. In the event a power bridge is
not available, oscillation can be detected by means of a crystal rectifier and .c.
milliammeter arrangement shown in Fig. 12. Adjusting the reflector voltage for a
maximum indication on the 0-1 milliammeter is about the most feasible way of getting
started. Further refinements will almost suggest themselves.
* Sperry Gyroscope Co., Trademark
* Pres., Joseph Racker Co.
† Chief Electronic Engr., Bogart Manufacturing Corp.
Posted January 31, 2019
|