If you are a Ham radio operator
- especially a DX (long distance) operator, you have been required to study and
learn about how the various layers of the Earth's ionosphere can, under predictable
conditions, be an excellent reflector of certain radio wavelengths, thereby facilitating
over-the-horizon communications. Reading this article is like a flashback from the
license preparation manuals - particularly for the General license exam. There is
a lot of information here. When this article was published in a 1958 issue of
Radio & TV News magazine, the world was nearing the end of the
International Geophysical Year (IGY), which had as its goal learning as much
as possible about the properties of the upper atmosphere. The first earth-orbiting
satellites were being launched and manned space flight was only months away, so
there was much interest in learning what could be expected while transitioning through
the ionosphere. It was during the IGY experiments that the
Van Allen radiation
belt was discovered.
An editorial entitled, "Scatter Communication," by editor Oliver Read,
appeared in the October 1955 issue of Radio & Television News.
Scatter Communication

The "Texas Tower" installation at top right makes extensive use
of scatter.
By Maurice P. Johnson
U.H.F. Communication Equipment Design Engineer
An ingenious method of transmitting signals far beyond ordinary line-of-sight
distances.
The advancement of the radio communication art is given impetus by the persistent
need for additional channel space as the spectrum fills with various services. In
addition, reliability of the communication link is becoming increasingly important.
Toward these ends, recent progress has been made in the development and application
of a new communication method, known as "trans-horizon" or "scatter" transmission.
This article is designed to acquaint the reader with the general principles of this
new communication technique.
Ground Waves and Sky Waves
The behavior of the radio waves which are radiated from an antenna is determined,
in part, by the operating frequency and by the angle of radiation. Energy leaving
the antenna along the surface of the earth is called the ground wave, while that
directed upward at an angle is known as sky wave.
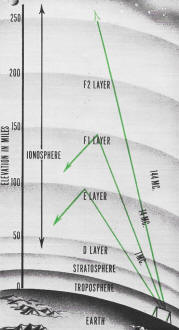
How ionosphere affects radio waves.
The ground wave is of interest because it is utilized by standard broadcast stations
in the 540 to 1600 kc. range. This ground wave blankets the local service area of
the station, but the signal strength dies out rapidly as the distance from the antenna
increases. The energy varies inversely as the square of the distance from the antenna
(free-space loss) plus additional attenuation due to absorption by the earth's surface.
As the operating frequency is increased, the losses become even greater, so the
ground wave is not useful for distant communication except at very low frequencies.
Let us then consider the sky-wave component of radiation. This wave would be of
little value were it not for the fact that the atmosphere acts to return some of
the energy to earth again.
The atmosphere may be considered as a series of layers of gases - primarily oxygen,
nitrogen, and hydrogen. The pressure and density decrease rapidly at distances removed
from the earth's surface. The "troposphere," the layer closest to the earth, is
responsible for weather and cloud formation and, most recently, is being utilized
for u.h.f. tropospheric scatter communication to be discussed later. Next is the
stratosphere, while at altitudes from 30 to 250 miles exists the layer of importance
to sky-wave radio communication, known as the ionosphere.
Some energy from the sun's ultra-violet radiation is expended in ionizing the
atmospheric gases to form the ionosphere. The various gases differ in their ability
to absorb this solar energy, so layers of ionization are formed. In order of altitude,
these layers are designated as D, E, and F. Since these layers are established from
the sun's radiation, it follows that they are subject to variation over the diurnal
cycle.
During the day, the D layer is found at an altitude of approximately 50 miles.
This layer is most intensely ionized at noon and disappears completely at night.
It does not reflect the sky wave, but it does absorb energy from, and thus attenuates,
signals passing through the layer.
At an altitude of approximately 80 miles is found the E layer, also a daytime
region, which acts to absorb the lower frequencies, but does reflect waves in the
2 to 10 mc. range back to the earth.
At altitudes of 150 to 250 miles, the interesting F layer is found. During daylight
hours, this may exist as two separate layers, F1 and F2, but at night the ionized
regions combine to form the single F layer. This layer is important because it is
the only ionized region which exists at night. Also, because the gas pressure at
this elevation is low, there is less variation in the layer from day to night.
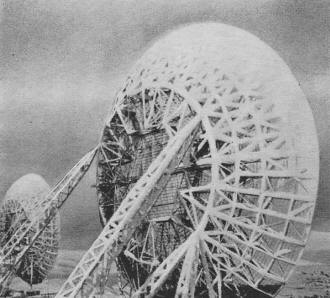
An antenna installation in Arctic Scatter Link. This type of
communication is being used extensively during the IGY tests.
Up to about 30 mc., signals passing through the E layer are reflected by the
F layer. Bending by the ionosphere is greatest at lower frequencies; as the frequency
increases a point is reached where the bending is insufficient to return the signal
to earth and the signal is thus lost to any earthbound receiving site. The distance
from the antenna to the point where a signal returns to earth is the "skip" distance
and is a frequency function. The transition between useful skip and lost signal
is the "critical" frequency. This varies diurnally. seasonally, and with sunspot
cycles, but generally occurs near 30 mc. Ionospheric skip has thus made possible
long-range communication in the 2 to 30 mc. range, so widely used by short-wave
broadcast, ham, and communication services. Higher frequency bands have been used
for line-of-sight paths, but this picture is now changing with the development of
scatter.
Critical and Usable Frequencies
As the ionosphere is constantly changing, skip propagation is not as dependable
or predictable as ground-wave coverage. Just as changes in the troposphere are studied
and predicted by the meteorologist, the ionospheric phenomena have been subjected
to study and forecast. Some terms used in this study are worthy of mention. The
"critical frequency" is the highest frequency which will be returned to earth when
a wave is directed vertically upward toward the sky. This is not necessarily the
same as the highest frequency which can be reflected back to earth if the wave is
directed at an angle toward the ionosphere. The "maximum usable frequency" (m.u.f.)
is the highest frequency which will be reflected back to a specific receiving location.
The m.u.f. may then be higher than the critical frequency because of the angle involved.
The "optimum working frequency" (o.w.f.) takes into account the possibility of the
m.u.f. changing during an operating period, by providing a safety factor to keep
ionospheric changes, weather, sunspots etc. from destroying the communication system.
Therefore, the o.w.f. is lower than the m.u.f. and is changed according to ionospheric
conditions. Thus, it becomes necessary to keep changing the o.w.f. in order to maintain
a reliable link over a given distance.

A rack-mounted receiver used for single-channel scatter circuit.
The equipment shown is the Type 1245 made by National Company. This and similar
gear is being used in both commercial and military experimental scatter communication
work.
Determining the w.f. would not be difficult if the ionosphere were not constantly
changing. The "normal" changes which may be expected, such as the change in virtual
height of the layers with time of day and season, may be predicted from year to
year with some accuracy. The critical frequency is also highest in years with large
sunspot numbers, in accordance with the eleven-year sunspot cycle. However, additional
"abnormal" variations may occur, such as meteor showers, sporadic-E ionization,
sudden ionospheric disturbances (s.i.d.) , solar flares, and polar blackouts due
to aurora and cosmic rays, all of which reduce the reliability of ionospheric skip
communication. While some ionospheric changes may be forecast, the many unpredictable
events which may completely block or destroy the signal make skip communication
networks far from dependable.
It might be thought that high-power ground wave would be more reliable, but it
is not the complete answer. This wave is so severely attenuated at the higher frequencies
as to be of no use. In the broadcast band, another difficulty presents itself. At
night the D layer disappears and with it the absorption so that now some sky wave
is returned to earth. At a receiving point, the phase difference between sky and
ground wave causes fading. Due to the frequency differences among carrier, upper,
and lower sidebands, there is frequency selective fading with resultant audio distortion.
This interference at the outer limits of the ground wave may actually reduce the
service area.
"Churns" and "Blobs"
In general, this was the status of the long range communication art prior to
World War- II. Tremendous development and progress in electronics came about because
of the war. In particular, radar work resulted in better tubes, high-gain antennas,
higher power high-frequency transmitters, and sensitive receivers with low noise
factors. These improvements opened higher frequency bands for radar, microwave links,
and television broadcast services. Microwave provided more reliable communication
systems than heretofore but was limited to line-of-sight distance per "hop." However,
as system performance was improved, some new aspects of propagation were discovered
and verified. Previous smooth-earth theory held that signals should attenuate very
rapidly beyond the horizon, for the usual line-of-sight-link. However, during and
after World War II, it was found that radar ranges were greater than expected in
the v.h.f. and u.h.f. bands. At first, this beyond-the-horizon signal was considered
an unwanted additional interference. In 1949, the "freeze" on new TV station construction
was put into effect because co-channel TV interference proved greater than anticipated.
Studies made by 1950 revealed that signals from 40 to 4000 mc. were stronger than
indicated by current propagation theory. Inversions, ducts, elevated layers, and
other theory could explain high signal levels, but investigations showed that the
signals were strong even when such phenomena did not exist.
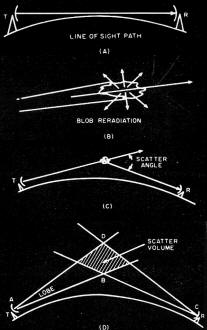
Fig. 1A - Simplified drawing illustrating the "line-of-sight"
path principle.
Fig. 1B - Signal re-radiation from "blob" is shown here and discussed
in the text.
Scalier propagation dish-type antenna. The car parked near lower rim of antenna
gives some indication of its tremendous size.
Fig. 1C - The scatter angle developed when signal is re-radiated
from the "blob."
Fig: 1D - The geometry of a signal path. It will be noted ABC
is shorter than line ADC.
The error was attributed to the assumption that the atmosphere was homogeneous
or that it varied in a smooth, gradual manner. It is now thought that the atmosphere
is in a constant state of turbulence and, as the medium "churns," eddies are formed.
This theory of turbulent areas, or "blobs," was advanced by 1950 and serves to explain
the medium of scatter. See Figs. 1A, B, C.
Frequencies above about 30 mc. are generally considered to be beyond the m.u.f.,
that is to say, they are not normally returned to earth in the conventional ionospheric
skip manner. To test the principles of scatter, experimental transmissions were
made with high power, over paths of several hundred miles between transmitter and
receiver, at frequencies in the 50 mc. range. Although this was above the m.u.f.,
a consistent but weak signal was received. This beyond-the-horizon link was possible
because of scatter, which we shall now discuss.
An Eimac 3KM50,000PA klystron mounted in a complete scatter amplifier. The magnetic
framework, external resonant cavities, and magnetic beam control coils are visible
in this close-up view photograph. High output powers can be generated.
Over line-of-sight paths, the transmitting and receiving antennas are aimed at
one another so that propagation takes place directly without using atmospheric reflection.
However, if the transmitting antenna is tilted up toward a "blob" in the atmosphere,
most of the energy will continue on through the blob and be lost to a receiver on
the earth's surface. But, according to a theory in physics (Huygen's wavelet principle),
this blob may be considered as receiving and re-radiating the signal. If we recall
the action of the parasitic elements of a yagi TV or ham antenna, the re-radiation
idea may be made clear. While most of the incoming energy will be re-radiated forward,
some small amounts of energy are re-radiated in other directions in varying strengths.
If the receiving antenna is directed at this blob, it will not be possible to receive
the energy that continues in the forward direction, but it will collect some of
the off-beam scattered signal which is of reduced strength. Over the path, the scatter
signal is thus greatly attenuated, but of great importance is the fact that a signal
may now be received at a point well beyond the horizon.
The transmitting antenna has a radiation pattern or lobe in the form of a beam
or cone-shaped volume. High-gain antenna systems may have a lobe narrowed to 5 degrees
or less in order to concentrate the power in the desired direction. In the region
of the atmospheric blob, however, the lobe has considerable cross-sectional area,
so that a number of blobs may be excited within the cone area. The receiving antenna,
aimed at this same region, "sees" a volume rather than a point in the atmosphere.
This volume is the "scatter volume."
Scatter Paths
Let us examine the path geometry diagrammed in Fig. 1D. It wrll be noticed that
the distance ABC is shorter than the distance ADC. The path length from transmitter-to-blob-to-receiver
will be different for each blob in the scatter volume. The lowest usable edge of
the lobes will be tangent to the earth, which is the shortest path distance, and
thus provides the strongest signal. Higher angle, longer path length result in reduced
signal strength because of increased free space loss plus reduced density in the
upper scatter volume.
The total received signal is the sum of the re-radiated energy which can be collected
from all blobs in the scatter volume. Differences in path distances within the lobe
cause delay differences in the multiple received signals. Less delay will be experienced
in narrow beams, but the attendant reduction in the effective scatter volume results
in consequent loss in total received signal.
In the broadcast band, differences in path lengths cause fading. In scatter,
the similar effect is known as multi-path fading or multi-path distortion. This
results in time and frequency distortion in the combined received signal. Time distortion
will lengthen or smear a transmitted pulse, while frequency distortion results in
frequency selective fading. The situation is further complicated by the constant
agitation inherent in the blobs within the scatter volume, which adds to the multi-path
fade problem. Thus, a normal characteristic of a scatter signal is a rapid continual
deep fade.
The path loss is great for a scatter signal and may be on the order of 100-150
db for an average distance. The loss is high because only a small part of the re-radiated
energy from the blobs is in such a direction that it can be collected by the receiving
antenna. Other losses may be encountered if antenna lobes do not cover the optimum
scatter volume for the distance involved. This is "antenna-to-medium coupling loss."
Also, an antenna made for high gain may be so large in terms of wavelength that
there will be phase changes over the aperture, resulting in some additional loss.
Thus it is found that for a given distance between transmitter and receiver,
there will be optimum values for beamwidth, scatter volume, and the scatter angle.
Despite multi-path distortion, severe rapid fades, and weak signal because of high
path losses, the distinct advantages of this propagation technique have made it
worthy of exploitation. System reliability is a prime factor, as will be seen, and
other advantages will become evident as the discussion continues.
V.H.F. and U.H.F. Scatter
It is now opportune to distinguish between the two types of scatter communication
that have been developed, namely v.h.f. and u.h.f. Above the m.u.f., scatter from
the ionosphere is utilized in the v.h.f. range of 30 to 100 mc. In particular, scatter
from the E layer is used in the 50 mc. band. This has resulted in reliable links
of from 500 to 2000 miles per hop. Experimental links in the v.h.f. bands have been
established in northern regions, as well as in the United States, to investigate
the effects of ionospheric disturbances on ionospheric scatter. Large rhombic antennas
were aimed at the E layer at path midpoint and recordings of carrier strength divulged
signal levels, signal-to-noise ratios, and fade effects. The experimental data confirmed
the continuous variation of the signal, with fade rates up to 10 cps. Supposedly,
this is due to multi-path effects as previously described. The fade may also be
quite deep, often more than 20 db from average.
Ordinarily, radio communication in arctic regions is difficult because of polar
blackouts, aurora, and other atmospheric disturbances. However, under circumstances
such that regular communication links are ineffective, scatter has proved to be
reliable and, in some cases, the signal has been enhanced by the disturbance. Scatter
is unaffected by many ionospheric disturbances, so a high degree of path reliability
is one of the very important values of scatter communication. Such v.h.f. scatter
links, above the m.u.f., may be of fixed frequency and yet provide a consistent
signal over periods-of years. Scatter from the F layer has not been satisfactory
for exploitation, but future work may disprove this. The E-layer, scatter does not
disappear at night as does ordinary ionospheric skip from this layer.
The channel space available in the u.h.f. bands is attractive, since in this
region wide-band communication may be realized. Thus, the second type of scatter
propagation has been developed in the range from 100 to 10,000 mc., using the "weather"
atmospheric layer for "tropospheric scatter." There is no effective ionization in
the troposphere, but there is variation in the dielectric constant and temperature
of the medium as the air pressure changes with altitude. Water vapor may vary the
dielectric constant, so the same "blob" theory of turbulence applies to this medium
of scatter. The tropospheric signal decreases rapidly with distance. The fall off
of signal strength with frequency above the m.u.f. is rapid for ionospheric scatter,
but is slow for tropospheric scatter. Tropospheric systems are therefore useful
for path distances of from 100 to 500 miles.
Fading Problems
We have already mentioned that a scatter signal is characterized by a "normal"
rapidly fluctuating fade due to the multiple signal paths in the scatter volume.
Additional fades have been attributed to Doppler frequency shifts resulting from
meteor trails, passing aircraft, or other moving objects in the path. Such fades
may vary from microseconds to several minutes duration and produce frequency and
amplitude variations in the signal. Slow fades are also experienced where the average
signal may change over periods of hours or longer.
Noise in the signal is generally due to cosmic rays or other solar sources. Aurora
is associated with another noise modulation, named "sputter" because of its distinctive
sound, resulting in a 200-300 cycle fade.
Because of the random variations of the fades, statistical studies have been
made of the signal path losses with some interesting results. Complete statistical
analysis takes into account Rayleigh and Gaussian probability distributions for
fade amplitude and duration. It is found that the chances of ever receiving a 100%
signal on the one hand and no signal (0%) on the other, are practically impossible.
With a given system and signal path and over a long period of time, a certain "statistical
average" or median signal-to-noise ratio will be found. The probability of signals
falling short of this value can be determined. It may be found that for 1% of the
time, fades of 20 db are experienced; and for 0.1 % of the time fades reach 30 db.
Thus, during these periods of time, fades will reduce the signal-to-noise ratio.
Fortunately, the heaviest fades are encountered for the smallest percentage of time
so that the minimum signal-to-noise ratio is found for only minimum time. For example,
a link may have a median signal-to-noise ratio of 25 db. For 1% of the time fades
may reduce this ratio to 11 db, and for 0.1% of the time, deeper fades may reduce
it to only 5 db. In practical terms, it is seen that in order for this system to
be reliable 99% of the time it must be capable of operating with a 11 db signal-to-noise
ratio. To increase system reliability to 99.9% requires operation from 5 db signal-to-noise
ratio.
Diversity reception provides some solution to the rapid fade problem. The signal
will seldom exhibit the same fade at two different locations at the same time. Additional
antennas, spaced some 50 to 100 wavelengths apart, have their signals combined.
High orders of diversity, that is combinations of several antennas, may reduce the
effects of rapid fades by adding the signals to a sum close to median level. This
space diversity (addition of antennas) may be combined with frequency diversity
(use of the same modulation on several frequencies) to combat the fast fade problem.
Ideally, diversity combiners increase the signal strength during fades, without
increasing the noise, so that signal-to-noise ratios are thereby improved.
By means of diversity, the signal can be recovered despite the fast fade introduced
over the scatter path. The result is a highly reliable propagation path which is
immune to the usual atmospheric disturbances.
Cover Story
Giant Telemetry Antenna
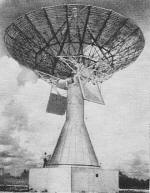
(Cover photo U. S. Air Force)
The giant telemetry antenna shown on this month's cover and in the photo above
is the first of five such units being scheduled for use in tracking ICBM's.
Constructed by Radiation, Inc., of Melbourne, Florida, the spider-web-like antenna
has been designated as the TLM-18 automatic tracking telemetry antenna. It is currently
undergoing tests at Melbourne (approximately 16 miles from Patrick AFB) prior to
its use by the Air Force.
Standing higher than a seven-story building, this huge piece of missile-tracking
equipment is 60 feet in diameter and is mounted on a steel tower 38 feet high and
20 feet wide. The tower houses the cables and driving mechanism which operate the
antenna at its required speeds. Data-recording mechanisms and remote controls are
located in separate, nearby buildings. The new antenna is designed to withstand
hurricane-velocity winds of up to 125 miles an hour.
Depending on a highly complex electronic operation, the telemetry antenna system
consists of a small radio transmitter aboard the in-flight missile. This transmitter
is equipped with a pickup device which samples such' phenomena as pressure, temperature,
acceleration, and control movement. Converted into electronic signals, the collected,
data is then transmitted continuously from the missile to ground.
Besides tracking intercontinental missiles, the TLM-18 is capable of tracking
the sun and other so-called "radio stars." This factor will make the antenna a valuable
asset in earth satellite experimentation during the current International Geophysical
Year.
The Melbourne unit is a predecessor to the four proposed missile-tracking devices
to be erected at selected sites. These new units will be built by the same company
upon completion of tests to determine the antenna's operational capabilities.
First of the proposed construction locations will be the Air Force Missile Test
Center's Cape Canaveral, Florida, launching site. Approximately 18 miles north of
Patrick AFB, the Cape Canaveral missile site consists of 14,513 acres of government-owned
land. It was transferred from Navy to Air Force jurisdiction on September 1, 1948.
A part of the Air Research and Development Command, the Air Force Missile Test
Center (AFMTC) operates the Florida Missile Test Range-the world's longest range
for testing guided missiles. The Center's mission includes conducting tests and
evaluating as well as collecting test data on guided missiles, controlled targets,
drones, and related equipment for the Air Force and, on occasion, for the Army and
Navy.
Besides operation of telemetry stations that are much smaller than the Melbourne
unit and which are located at numerous islands sprinkled throughout the Atlantic,
ARDC also has at its dispose] a chain of floating bases that currently bridge about
3000 miles of ocean. Timed to coincide as closely as possible with missile fests,
a fleet of re-commissioned World War II and Korean freighters are being used by
ARDC to pick up and transmit data to the Cape Canaveral Center.
Three other proposed sites for construction of the giant telemetry antennas include
the Missile Test Center facilities on Antigua, B.W.I.; Fernando de Noronha Island,
off the east coast of Brazil; and Ascension Island in the south Atlantic. The four
antennas will cover the entire 5000 mile distance of the range with a moderate overlap.
The new telemetry antennas thus will supersede existing tri-helix antennas at the
Cape and several of the auxiliary island stations. The older antennas, which provide
manual trading, will be retained for back-up use.
Equipment Requirements
Several requirements dictate the design of equipment for scatter. With a high
path reliability to exploit, equipment should be extremely reliable, to insure dependable
communication links. Because of the weak signal over the path, equipment must operate
from minimum signal-to-noise ratios, with low noise factor and high sensitivity.
For efficient spectrum utilization, multi-channel modulation is used to permit transmission
of many telephone, teletypewriter, or facsimile signals simultaneously.
Multi-channel modulation is accomplished by "multiplexing" techniques. Frequency-division
and time-division multiplex are the two methods most commonly used. Time-division
multiplex may be likened to a commutator-type rotating switch which samples any
particular signal circuit for only a fraction of the total time. Bits from each
signal circuit share a common modulation channel, separated by time intervals. Only
a part of the total signal information is utilized in the multiplex channel but,
in many cases, the sampling rate is fast enough to collect adequate information.
Time-division multiplex requires minimum bandwidth and is used for modulating
v.h.f. scatter systems, which have little spectrum space available. Many ingenious
methods of adapting this multiplex technique have been devised to fit the available
band-width and to function with low signal-to-noise ratios.
With wide-band modulation avail-able over u.h.f. scatter links, the wider frequency-division
multiplex may be exploited. Fig. 2 illustrates the "base-band," which is the audio
spectrum up to say 300 kc., divided into channels for individual signal circuits.
Each channel is shown as 4 kc. wide, with 0.2 kc. guard space between the channels.
Note that the space from 200 to 4000 cycles has been reserved for "order-wire,"
which is a telephone circuit used for technical adjustment and test of the scatter
link. Usual multiplex gear will produce multiples of twelve channels, so a typical
base-band may contain 12, 24, 48, or 72 channels. The individual signal circuits
are injected into the baseband in the proper spot by a process of heterodyne frequency
conversion. This may be compared to the action of a superhet receiver which converts
the incoming signal into an i.f. signal by beating against a local oscillator. For
base-band, the function is to "heterodyne up," since the final baseband frequency
is usually higher than the incoming signal. This multiplex action permits many signals
to share a base-band by frequency separation of channels. Frequency-division multiplex
requires a wide modulation channel, but permits all intelligence in each channel
to be simultaneously transmitted.
Thus, by multiplex methods, a scatter link can efficiently carry many narrow-band
signals simultaneously or wide-band signals, such as television, may be transmitted
over the wide-band modulation circuits of u.h.f. scatter systems.
Actual methods of modulation for scatter systems are currently being investigated.
Both AM and FM have been utilized, with SSB showing definite advantages in many
respects. System performance in the face of noise is of extreme importance. The
threshold effect of FM seems to indicate the SSB will be superior at low signal-to-noise
ratios. Theoretical investigations indicate that SSB is superior in reducing bandwidth,
operating at low signal-to-noise ratios, and under multi-path fade conditions.
Designers are overcoming the problems of developing SSB scatter equipment for
the u.h.f. bands. For example, sideband information must bear a definite relation
to the carrier for most SSB receiving systems to operate, since a carrier must be
re-inserted to recover the SSB modulation. Frequency shifts and fades over the scatter
path may destroy this relation, if the carrier is completely suppressed. However,
if greatly reduced pilot carriers are transmitted in the SSB system, these pilots
may be used for recovery of the modulation without distortion.
With only one sideband transmitted, the SSB system requires considerably reduced
bandwidth for a given amount of intelligence, as compared to FM. It also requires
less transmitted power than other methods of modulation, which is an important factor
in view of the very high transmitter powers used for scatter links.
Complete Systems
An idea of the complete scatter system may be gleaned from a description of the
equipment. A typical v.h.f. scatter link might make use of high-gain antennas, separated
for diversity reception. Antenna gains of over 20 db are possible with yagi, bedspring,
corner reflector, or rhombic antennas measuring as much as 1000 feet per side. Special
transmit-receive filters are included to permit the common antenna system to function
for transmission and reception. The main and standby transmitters may well be 10
or 20 kw. installations in the 50 mc. band. Such transmitters may be similar to
the high-power v.h.f. equipment used in TV broadcast service. High power output
tubes, such as the 6166 may be used, with plate voltages above 5000 and plate currents
of several amps. The exciter may be low power, utilizing balanced modulators and
other SSB techniques. The tolerances will be found to be stringent, with distortion,
intermodulation, and phase shifts kept within microscopic limits. In some systems,
frequency stability of oscillators may be kept within one part in 100 million. Such
exacting specifications are needed for certain types of SSB multiplex work.
Receivers are designed for minimum noise figure, high sensitivity, and minimum
intermodulation distortion. Oscillators again are extremely stable. Accessory equipment
is used to convert recovered multiplex into original teletypewriter or other signal
intelligence.
Maximum equipment reliability is obtained by conservative design, complete spare
transmitters and receivers, and rapid change-over facilities. This reduces the out-of-service
time to a minimum.
For a u.h.f. scatter system, the antennas will usually be huge, specially designed
parabolic dish reflectors fed by waveguide horns. Such antennas may be 28 or even
60 feet in diameter, with ingenious design to provide mechanical strength and minimum
warpage with wind and icing conditions.
Diversity will again be found, with suitable microwave diplexing techniques utilized
for transmit and receive. Power may be 10 kw. or more at frequencies of 1000 or
2000 mc., using huge multi-cavity power amplifier klystrons as output tubes. Bandwidth
and linearity are design criteria and distortion is kept to very low levels. Exciters
may use FM or SSB with multiplex or TV modulation.
Receivers use microwave crystal diode cavity mixers and traveling-wave tube preamplifiers.
Cascade and low-noise i.f. amplifiers, often with double conversion, follow the
microwave mixers.
In general, it may be seen that permanent scatter installations are rather elaborate
and complex. The antenna installations are engineering achievements in themselves.
Including spare and standby equipment, a scatter installation may be the size of
a high-power AM broadcast station. However, recent work has been directed toward
producing portable scatter equipment, in particular for military tactical use. Systems
of one kilowatt power or so have been mounted in truck-trailer vans, completely
mobile with demountable antennas and primary power plants. Installations have been
designed featuring inflatable antenna systems. Such equipment may be moved in cargo
aircraft or by helicopter, ready for tactical use.
A comparison between a scatter link and a line-of-sight link is of interest.
The over-all path loss of the scatter link may be 150 db or more while a typical
line-of-sight path may have a path loss of 100 db. Although the link distance is
greater for scatter, it is seen that some 50 db greater gain is needed for the scatter
link. This is accomplished by higher power in the transmitter, probably 10 kw. as
compared to 10 watts and an antenna gain of as much as 35 db as compared to 15 db.
Alignment of the scatter link is difficult because the two sites are beyond line-of-sight.
The great circle path is used and surveying must be extremely accurate. Final alignment
is for maximum signal strength. Path losses are often difficult to predict or calculate.
The actual received signal may be -100 to -150 dbw. The received signal may fade
into the noise level at times, but the diversity action previously discussed permits
reliable operation at very low signal levels.
In comparison, it may be said that multi-path distortion and the limited spectrum
space reduce the bandwidth possibilities of v.h.f. scatter systems, but hop distances
of 500 to 2000 miles are possible. The u.h.f. scatter is limited to less than 500
miles per hop, but delay distortion is not as severe and spectrum space is available
for wide-band modulation. Thus tropospheric scatter will provide facilities for
multi-channel multiplex and TV circuits. The multi-channel possibilities make u.h.f.
economical for expanding telephone and television net-works.
Applications
The military and commercial applications of scatter equipment have spurred development
and installation. Extensive scatter links are planned and being installed. Many
military installations are classified, but it is common knowledge that the "Texas
Tower" radar stations make use of scatter communication links, as do the DEW and
other arctic early warning radar lines. Other military networks are being installed
in North Africa and Europe.
Commercially, telephone and television interests see obvious advantages in scatter
for extending and expanding present communications systems. Because of the increased
separation possible between the stations, new links are practical. Over-water island
hops are now possible and several are already in operation (between Florida and
Cuba and between Puerto Rico and Dominican Republic). Several others are being planned,
The future may find more countries and even continents interconnected with TV and
communication scatter networks.
Additional propagation tests are planned, particularly during this International
Geophysical Year, including tropical path studies. Transatlantic scatter systems
are planned, in particular connecting Canada, Greenland, and the United Kingdom,
This proposed scatter link at 30-45 mc. for voice and teletypewriter will do much
to overcome the existing frequent blackouts in radio teletypewriter circuits. Transatlantic
television links may be the next step, Truly, the future of scatter communication
is "beyond the horizon."
Posted October 15, 2019
|