August 1969 Electronics World
Table of Contents
Wax nostalgic about and learn from the history of early electronics. See articles
from
Electronics World, published May 1959
- December 1971. All copyrights hereby acknowledged.
|
Francis A. Gicca, manager
of Raytheon's Space Communications Systems, published a very extensive two-part
article in Electronics World magazine in 1969. Part 1 covered
Score
through Intelsat II
satellites which launched between from December 1958 and December 1968, respectively,
in the July 1969 issue. Part 2 begins with
Intelsat III,
which commenced operation in September 1968. Rather than reiterating the article's
contents, I will offer an anecdote about the altitude used by geostationary satellites,
which is 22,300 miles. In the early 1990s, I worked for a few years at
COMSAT Laboratories
(Communications Satellite Corporation, famous for involvement in both Intelsat and
Inmarsat), in Clarksburg, Maryland. The mailing address there was 22300 Comsat Drive.
I just looked for my Inmarsat/Comsat project member coffee mug and cannot find it,
I'll post a photo of it when I do.
Communications Satellites - Success in Space
Part 2; Operational Satellites of Today and Tomorrow
By Francis A. Gicca, Manager Space Communications Systems, Raytheon Co.
Inaugurating a real revolution in the field
of communications, our successful satellites promise even more startling advances
in the near future. Description of the present Intelsat system, along with uses
of satellites for aeronautical communications as well as domestic television and
for telephone services.
Part 1 of this series traced the early history of communications satellites from
Score to Intelsat II. All of these satellites were designed to be experimental but
all provided valuable operating experience and confidence in communications satellites.
This concluding article will examine the operational satellites of today and tomorrow.
Early in 1966 Intelsat awarded a $32-million contract to TRW Systems for six
Intelsat III satellites, which were intended to provide the Global System envisioned
by the Interim Agreement (see Part 1). Four times as heavy as Early Bird and with
five times its capacity (see Table 1), Intelsat III is the first satellite specifically
designed to be commercially profitable. For its first operational system, Intelsat
attempted to maximize all satellite parameters that would affect revenue; including
radiated power, usable bandwidth, capacity, and life.
Power radiated at the earth is maximized through the use of a de-spun antenna.
As indicated in Part 1, this approach counter-rotates the antenna beam from the
spin-stabilized satellite body thereby keeping the beam pointed at earth. Originally,
Intelsat III was to use an electrically de-spun array, but a mechanically de-spun
antenna was later substituted (see Fig. 1). As in NASA's experimental ATS-1,
the electrical system proved to be lower in effective gain than the mechanical.
Experience with OSO, ATS, and other NASA satellites with rotating parts indicated
that reliable operation could be expected for more than five years. It was originally
feared that the lubricants needed would either freeze or evaporate in space and
bearings would seize. Special lubricants and lubricating techniques were developed
to operate in the zero-G vacuum environment and have proved very successful. De-spun
platforms weighing several hundred pounds are being designed into to day's satellites.
Intelsat III Repeater

Table 1 - Important characteristics of the entire series of Intelsat
communications satellites.
The total usable bandwidth of Intelsat III is 450 MHz, supplied by two 225-MHz-wide
repeaters. This is a utilization of 90% of the 500-MHz bandwidth currently allocated
for communications satellite use. The de-spun earth-coverage antenna and 6-watt
traveling-wave-tube (TWT) amplifiers produce a radiated power of +22 dBW (158 W)
from each repeater. This power and bandwidth are suitable for four TV channels,
or two fully loaded carriers with 1200 telephone channels each, or up to 90 multiple-access
carriers each with 24 telephone channels, or any equivalent combination of traffic.
While Early Bird was designed for an operating life of 1 1/2 years and Intelsat
II for 3 years, Intelsat III has a specified minimum life of 5 years. This represents
almost a twenty-fold progressive increase in circuit-years from 1965 to today. This
increase has been possible primarily because of the growing confidence in the reliability
of communications-satellite components. While Early Bird was designed for a lifetime
of only 18 months, it is still operating satisfactorily after four years. The next
generation communications satellite (Intelsat IV) will have a projected life of
70,000 circuit-years, a hundred-fold increase over Early Bird.
A simplified block diagram of Intelsat III's communications section is shown
in Fig. 2. Communications signals from earth stations arrive at the satellite
within either of two 225-MHz-wide bands; 5930-6155 MHz or 6195-6420 MHz. This leaves
two outer 5-MHz guard bands and a central 40-MHz guard band which also contains
redundant satellite-command signals.

Fig. 1 - Intelsat III employs a mechanically de-spun antenna.
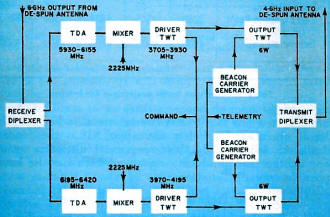
Fig. 2 - Simplified block diagram of the Intelsat III.
After reception by the de-spun antenna, a filter-diplexer separates the signals
into the two repeater channel bands. Linear r.f. amplification is accomplished by
a series of low-noise wide-band tunnel-diode amplifiers (TDA's). An input of -65
dBm to the TDA's will produce a saturated TWT output of 6 watts; a net repeater
gain of +103 dB.
The signals are then down-converted by 2225 MHz in a wide-band mixer. The two
channels that are produced are now in the downlink bands of 3705-3930 MHz and 3970-4195
MHz.
The principal r.f. amplification is now accomplished in a cascaded pair of driver
power TWT's. After each driver TWT, the redundant command signals are filtered out
and removed from the communications channel. Telemetry signals are phase-modulated
onto two beacon signals which are then inserted into the central 40-MHz guard band
just, prior to the 6-watt power TWT's. These redundant beacon signals allow the
earth-control stations to track the satellite while it is being injected into orbit.
After amplification to the 6-watt level, the two repeater channels are recombined
in the transmit diplexer and transmitted by the de-spun antenna.
The block diagram of Intelsat III is deceptively simple. For the satellite to
be efficient its capacity must be limited only by its radiated power and its effective
utilization of bandwidth. The former is generally limited by the weight (and thus
cost) of the satellite, and the latter by bandwidth allocation constraints (500
MHz at present). This implies that the system cannot economically tolerate any significant
degradation of quality by the satellite. This, in turn, means that all forms of
repeater distortion must be exceedingly low, typically 56 dB signal-to-distortion
ratio, and this is far from simple in such a wide-band repeater.
Principal sources of distortion in the repeater include: 1.intelligible crosstalk
(any amplitude variations in the repeater convert some of the FM modulations to
AM, and the TWT converts these back to FM which intelligibly interfere with the
original signal), 2. delay distortion (caused by lack of phase linearity which delays
some frequencies more than others, causing a distorted signal), 3. intermodulation
between multiple carriers (caused principally by the amplitude non-linearity of
the TWT's plus those in the earth stations), and 4. amplitude crosstalk between
multiple carriers (multiple carriers produce a composite signal of varying amplitude,
the AM-to-PM characteristic of the TWT converts this to an interfering signal).

An Intelsat III, with capacity of 1200 voice circuits, is shown
here in test chamber. Satellite was successfully launched on Dec. 18, 1968 and placed
in synchronous orbit over Atlantic from where it is providing commercial service.
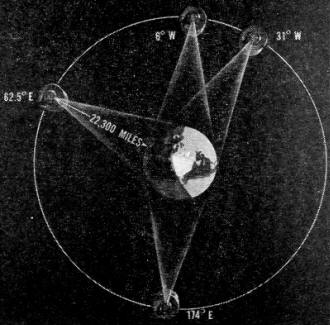
The Intelsat III global satellite system. Two (6°W, 31°W) are
shown over Atlantic, one (174°E) over Pacific, and one (62.5° E) over Indian Ocean.
Two are now in orbit, other two will be orbited shortly.

Looming over nearby fields at Cayey, P.R. is this 97-ft parabolic
antenna for satellite communications.
As a result, unless extreme care is taken, all elements of the repeater can produce
major distortion. The microwave filters used for diplexing must produce extremely
high rejection out-of-band (to eliminate signals that might cause intermodulation
and amplitude crosstalk), but must be very flat in-band (to prevent intelligible
crosstalk) and linear in phase (to prevent delay distortion). The TWT's have to
be efficient (which means saturated operation) but must have a low AM-to-FM characteristic
(which requires linear operation far below saturation). It is the repeater designer's
job to trade these sources of distortion against one another and produce a repeater
which has the lowest possible over-all distortion.
The Network Goes into Service
The first Intelsat III was launched on September 18, 1968. Shortly after lift-off
the guidance system of the long-tank Delta malfunctioned and the launch vehicle
was destroyed by the range safety officer.
The second launch took place on December 18, 1968 and successfully placed an
Intelsat III at 31° west longitude over the Atlantic. Three more Intelsat III
satellites will be at 174° east longitude (Pacific) (already in orbit), 6°
west longitude (Atlantic), and 62.5° east longitude (Indian). All are to be
in place by the end of this year thus completing the first global system (see Fig. 3).
This system of four satellites will be able to handle a maximum of 4800 two-way
telephone circuits or sixteen TV transmissions.
The standard earth station that completes the Intelsat III global system is an
impressive terminal. All standard stations have an antenna at least 85 feet in diameter
and many are larger.
Typical of the Intelsat earth stations is the new Comsat station at Cayey, Puerto
Rico. The antenna, landmark of the station, stands taller than a 10-story building.
Total weight of the Cassegrain antenna is about 470 tons. The surface of the reflector,
97 feet in diameter and weighing 45,000 pounds, was designed to an accuracy of better
than 50 thousandths of an inch. The antenna and receiver were verified to meet the
40.7-dB gain-temperature sensitivity requirement by listening to the known radio
emissions of the star Cassiopeia A.
To meet the required receiver noise temperature of 50° K, liquid-helium-cooled
parametric amplifiers are housed in an equipment room behind the reflector. In operation,
the earth station tracks any small motions of the satellite to 0.04°, using
an auto track system which is locked to the satellite beacon.
The Cayey station is one of six in the United States; Andover, Maine; Brewster
Flat, Washington; Paumalu, Hawaii; Jamesburg, California; and Etam, West Virginia.
By the end of this year 44 earth stations are expected to be in operation. By 1972,
the number is expected to rise to 86.
If these stations should each transmit only 100 telephone calls through the satellite,
the capacity of all Intelsat III satellites would be exceeded by 80%.
Multi-Purpose Satellites
Because of the great expansion in the number of earth stations, it became clear
early in 1967, two years before the first launch of Intelsat III, that the satellite's
capacity probably would not be sufficient to meet eventual traffic demands. Thus,
on March 23, 1967 two study contracts were awarded to investigate an advanced global
satellite over three times as heavy as Intelsat III and capable of multiple communications
missions, including domestic and mobile communications as well as international
traffic.
Two types of stabilization were studied for this 2000-pound Multi-Purpose satellite;
the familiar spin stabilization and a fully stationary satellite approach. In the
latter, the vehicle is stabilized by an internally spinning inertia wheel and the
satellite body is stationary. In effect, the entire satellite is "de-spun" with
the internal inertia wheel supplying the necessary gyroscopic stability. This approach
is interesting because all moving parts are in a sealed inertia wheel case and thus
should be more reliable. The antenna and repeater are rigidly connected to the rest
of the satellite thereby avoiding slip rings and power transfer joints, and solar
paddles, which are pointed at the sun to increase the communications power available,
can be used. Although Intelsat eventually decided this approach had too many technical
risks for immediate commercial use, it is probable that future satellites will be
fully stabilized. Europe is presently developing an experimental communications
satellite, called Symphonie, which will use the inertia-wheel stabilization approach.
Maximizing Capacity

Table 2 - Telephone traffic projections for Intelsat.
Because of the Multi-Purpose satellite's great weight, it became possible, perhaps
for the first time, to seriously consider how this weight could be utilized to maximize
the communications capacity of the satellite. Previous satellites were weight-limited
to the point where such optimization was really not possible.
In retrospect, a principal deficiency of the Intelsat III design lies in its
extremely wide repeater bandwidths for multiple access. Since multiple users must
therefore share the same repeater, the TWT must be operated far below saturation
to avoid distortions previously mentioned. This, in turn, is an inefficient use
of the satellite's power and causes serious reduction of total capacity.
Another disadvantage of wide bandwidths is the requirement that each earth station
accurately control the power that it sends to the satellite. Since a satellite's
repeater power will be distributed among all users in proportion to the strength
of the received signals, an improperly large signal from one earth station can capture
all the repeater's power. To avoid this situation, each station must accurately
monitor its power and control it in proportion to its traffic needs. This is both
difficult and impractical. Experience with Intelsat III has shown that stations
cannot easily maintain the required accuracy.
These considerations suggest that the available bandwidth should be divided into
many little repeater bands and each earth station assigned its own exclusive repeater
and saturated TWT. This is not entirely possible since each band must be set at
least as wide as the widest bandwidth signal to be carried and it is likely that
several users might still wish to multiple-access that band with small traffic.
Still, this approach is a great improvement.
As might be expected, the widest bandwidth user is television, requiring a band
of about 40 MHz in the Multi-Purpose configuration. Twelve such bands fill the available
500-MHz bandwidth. Twelve 8-watt or 12-watt TWT's would completely use the 2000
pounds available, depending upon whether complete electronic redundancy is used
or not. Since the capacity of the Multi-Purpose satellite is affected more by bandwidth
limitations than power (it is bandwidth-limited), the lower power redundant configuration
was chosen because of the significant increase in circuit-years for this choice
(a lifetime of 7 years). With a repeater bandwidth of 40 MHz, 8-watt power, and
earth-coverage antenna, each of the 12 repeaters can handle 1800 telephone channels
or one TV channel.
Start of the Fourth Generation

Fig. 3. The 1970 Intelsat III global system will consist
of four satellites and a large number of ground stations. This entire system will
be able to handle a maximum of 4800 two-way telephone circuits or 16 TV transmissions.
By early 1968 international traffic projections were similar to those shown in
Table 2. It was becoming clear that by 1971 Intelsat III's capacity in the Atlantic
and Pacific would be exceeded. Furthermore, should any satellite fail, the system
would be in serious trouble; adequate Intelsat III in-orbit reserve capacity did
not exist and Intelsat I and II would be well beyond their design lives. After first
considering the purchase of additional improved Intelsat III satellites (Intelsat
III 1/2), it was decided to proceed with the Multi-Purpose international design,
which was named Intelsat IV.
A $72-million contract for four of these giant satellites was awarded to Hughes
Aircraft last fall with the first two launches scheduled for early 1971 (Atlantic
and Pacific).
The third satellite will be placed over the Indian Ocean in 1972 (at Intelsat
III end-of-life) and the fourth satellite will be kept as a spare.
In addition to its earth-coverage de-spun antenna beam, Intelsat IV will also
have two 4.5° spot transmit beams that can be aimed at high traffic points on
the earth. Eight of the twelve 40-MHz repeaters (36-MHz usable bandwidth) can be
connected to the spot beams, increasing their effective radiated power 15 times.
The maximum capacity of Intelsat IV will be over 10,000 two-way telephone calls
or twelve TV channels with greatly improved access.
Aeronautical Communications
The Multi-Purpose study also was the first to investigate the use of satellites
to communicate with aircraft. At present, transatlantic flights are out of range
of shore-based v.h.f. communications for about 90 minutes. It is projected that
during this year's August transatlantic peak, 85 aircraft will simultaneously occupy
this 1000-mile-long "radio-silence" corridor in the North Atlantic. At about the
same time, 40 aircraft will be out of radio contact over the Pacific as will 10
aircraft over the Indian Ocean.
Artist's concept of fully stationary Multi-Purpose communications
satellite. Large antennas provide steerable spot beams for high-traffic areas. Entire
vehicle remains pointed at earth at all times.
Mockup of giant 2000-lb Intelsat IV, scheduled for service in
1970's. It has capacity of up to 10,000 telephone calls or 12 color-TV broadcasts.
Several proposals have been made for the use of satellites for
U.S. domestic distribution of TV and telephone calls. Satellites would either blanket
U.S., as shown, or use spot beams to provide time-zone coverage.
This lack of communications is the principal reason for a required lateral separation
of 120 miles between aircraft over the Atlantic. The restriction is expensive to
the airlines because it prevents them from operating flights when passengers would
like to fly, and forces them to schedule to meet the separation requirement. In
ten years the transatlantic peak in the silent corridor is expected to exceed 150
aircraft and will consist of a mix of supersonic, jumbo, and conventional jets.
To make this high density possible, lateral separation must be reduced to below
60 miles. If a recommended minimum safety criterion of less than one collision in
100 million flights (one incident per thousand years) is to be met for this separation,
the position of each aircraft in the corridor must be known to better than 15 miles.
Such accuracy requires that each aircraft be under surveillance at all times, and
this can he done only by satellites.
Simplest would be an aeronautical comsat (aerosat ) that interrogates aircraft
for position information as well as air traffic control communications. An advanced
approach would also verify aircraft position, using a ranging code.
From the airlines' point of view, the present 118-136 MHz v.h.f. aeronautical
band would be ideal for such an aerosat since it would mean the least change. The
v.h.f. experiments between ATS-1 and test aircraft over the Pacific, and Air Force
experiments with LES have proved that a v.h.f. aerosat can be built today. The principal
obstacle is that v.h.f. is not allocated for satellite use and government officials
are reluctant to risk further possible interference in this congested band.
A possible alternative is the use of the aeronautical L-band (1.540-1660 MHz)
and the FCC has recommended that this band be used by aerosats on a shared basis.
Since aircraft equipment is not currently available at L-band and practical aircraft
antennas do not exist, airlines have not been anxious to proceed. Instead, the airlines
are arguing internationally that a portion of the v.h.f. band should be allocated
for this service and that L-band should be developed gradually. In any event, because
of the urgent need, it is probable that some form of aerosat will be under development
before 1970.
Comsats for Domestic Applications
But perhaps the most significant portion of the Multi-Purpose study was that
concerned with the design of domestic comsats. If comsats have had such an impact
on international communications, why not use such satellites for domestic transcontinental
traffic?
It all started in 1966 with FCC Docket 16495 which was established after ABC
and the Ford Foundation both filed for authority to orbit television-relay satellites.
ABC applied on the basis of cost savings to distribute its TV programs, while the
Ford Foundation argued that similar savings could help support educational TV. These
applications were quickly followed by others including two from Comsat and AT&T,
who argued that any domestic satellite should be designed for both TV and telephony.
All proposals are still being evaluated by the government, for as FCC Chairman Hyde
has stated, "No Commission task is more challenging ... than the determinations
to be made concerning the proposed use of satellites for domestic purposes."
The potential impact of these proposals also caused President Johnson in 1967
to establish a Presidential Task Force to review the entire national communications
policy. This task force, under Presidential advisor Eugene Rostow, made its unreleased
recommendations to President Johnson last December and it is likely that major reforms
in the Communications Act of 1934 are being considered.
Although the value of distributing transcontinental TV signals via satellite
is clear, the domestic distribution of telephone signals will ultimately prove to
be a most significant development. At the moment, a domestic satellite would provide
better transmission quality than any ground link longer than about 1500 miles. This
is so because a satellite's signal quality is essentially independent of distance,
while a terrestrial link deteriorates directly with distance. Further, since satellite
cost compares favorably with ground links, there is little reason to develop new
long-distance microwave ground links. However, traffic projections for the early
1970's show a need for additional transcontinental capacity. All these factors favor
the early development of a domestic TV/telephony satellite.
In time, this situation is likely to produce more reliance on satellites and
less on ground links. By the early 1980's, large-capacity domestic satellites could
be handling messages over distances beyond 500 miles to open a new generation of
inexpensive public communications.
In December, 1966 Comsat proposed to begin domestic service with a Pilot satellite
designed to provide practical operational experience prior to the establishment
of a full system. The Pilot satellite is essentially a 12-channel Intelsat IV satellite
with the earth and spot beams replaced by a 3.5° X 7.5° beam that blankets
the U.S. The Pilot system would employ two such satellites, two east-coast and two
west-coast Intelsat-quality access stations, and about 30 midwest receive-only TV
terminals (32-foot antennas).
Interest in domestic satellites is not confined to the United States. France
has studied a domestic satellite (Saros ) to connect continental France to colonies
in the Caribbean and Africa. It has also studied a radio-TV satellite to link France
and French-speaking Quebec in Canada. West Germany has studied a domestic satellite
(Olympia) and proposed its use for TV distribution of the German Olympics in 1972.
France and Germany agreed last year to join forces and construct a common satellite
(Symphonie) to accomplish the previous Saros/Olympia missions. This satellite is
under construction and scheduled for launch by 1972. Canada has just concluded two
studies of a domestic satellite to help it distribute radio, TV, and telephony to
previously isolated communities in the northern Provinces. India and Brazil are
also considering domestic systems. Even with its relatively small area, Japan has
studied the development of its own system, as has the State of California.
The operational successor to the Pilot satellite is likely to employ four 2°
spot beams to illuminate the four U.S. time zones for more versatile TV distribution.
This raises the interesting possibility of re-using the same 500-MHz down-link frequencies
in every other time zone. The selectivity of the spot beams would keep these same
frequency signals from interfering with each other. This would essentially double
the TV capacity of the bandwidth-limited satellite to 20-24 channels. Since most
programs originate at either Los Angeles or New York, the problem is how to transmit
up to 20-24 channels on the single available 500-MHz up-link band. This number of
channels is required on fall weekends when the ABC-CBSNBC-NET networks are telecasting
different programs in each time zone plus regional football games.
Frequency Allocations and the Future
It is apparent that the above problem is a symptom of insufficient available
bandwidth. At the end of next year, the ITU plans to convene a World Administrative
Radio Conference (WARC) which will consider, among other things, the allocation
of additional frequencies for communications satellite use. Table 3 shows present
and proposed frequency assignments. The two 500-MHz bands currently allocated at
4 and 6 GHz are shared with terrestrial microwave links. This has led to specific
restrictions on satellite power levels so that they will not interfere with established
microwave links. The same situation is true for the military band, but the problem
here is not as severe since the traffic needs are considerably less.

Table 3 - Present and proposed frequency assignments for comsats.
In preparation for the WARC, the FCC recently recommended additional assignment
of two 20-MHz bands at L-band for mobile aerosat use, and two additional new 1000-MHz
bands exclusively for communications satellite use. Although the new point-to-point
bands would triple the currently available bandwidth, the new frequencies are in
the millimeter band where they are severely attenuated by rain. For reliable operation,
several widely spaced redundant earth stations might be required at each site so
that one would always have favorable propagation. This is expensive and so Comsat
would like, and has requested, the additional allocation of shared frequencies at
X-band on an "as available" basis. Comsat has also requested that the v.h.f. aeronautical
and marine bands be shared with an aerosat.
Although there exists justifiable economic reluctance to begin development of
a millimeter band for communications satellites, the use of this band is inevitable.
Communications satellites have become popular candidates for many domestic applications
and the pressure for exclusive frequencies for advanced domestic applications is
great. Only in the millimeter region is exclusive allocation possible.
Not only has this portion of the spectrum room for a fantastic amount of communications,
it also offers the hope of extremely inexpensive world-wide communications. By the
turn of the century it is quite likely that most industries and many homes will
have direct millimeter-wave access to communications satellites. This will not only
allow allow most instant global connections, but will also allow the centralization
of facilities that are now too expensive to duplicate at several locations. It will
start the era of centralized world libraries with computer-aided searching and abstracting,
global facsimile transmission, automated medical diagnosis using centralized case
storages, home tele-shopping with individual televiewers and banking units that
debit our account when we place an order, as well as direct communications between
computers to form fantastic control systems. Also operating will be huge broadcast
satellites that can transmit radio and TV programs directly into the home. Considering
what communications satellites have achieved in the past ten years, the only real
question is whether it will take as long as 30 years for this next revolution to
happen.
The author would like to thank the Communications Satellite Corporation, NASA,
and the U.S. Air Force for their kind help in preparing these articles..
Posted May 24, 2024 (updated from original post
on 8/21/2017)
|