January 1963 Electronics World
Table
of Contents
Wax nostalgic about and learn from the history of early electronics. See articles
from
Electronics World, published May 1959
- December 1971. All copyrights hereby acknowledged.
|
It seemed weird to read of microelectronics device density expressed
in parts per cubic foot of semiconductor substrate. Describing
density that way makes some sense when considering 3-dimensional
devices with vertically stacked elements, but this was in a
1963 article in Electronics World, so that could not
have been the case. The motivation, evidently was to be able
to compare microcircuit density with that of the human brain
in terms of neuron density. In fact, there is an interesting
chart presented that shows the evolution in circuit density
beginning with vacuum tube circuits, progressing through the
state of the art in 1963, projecting for future years, and finally
peaking with the brain's density. Interestingly, the brain density
shows as about 5x1011/ft3, while the "nonredundant
semiconductor device" limit is 1013/ft3.
Contemporary measurements estimate a neuronal density of around
4x104/mm3 (1x1013/ft3),
which is about a factor of 10-20 over a span of 50 years
(not too bad). I gave up trying
to find useful data on current transistor density in volumetric
units, but in terms of devices per mm2,
Intel is running better than 30 transistors/mm2,
which, although not really a legitimate exercise, translates
to [√(30 transistors/mm2)]3 = 2x102
transistors/mm3. That means we're now at about 1/200th
of the human brain density of 4x104 neurons/mm3.
Microelectronics
By William O. Hamlin / Fairchild Semiconductor
The
electronics industry is on the verge of a new era with the introduction
of integrated microelectronic circuits. This new concept, now
in active development by a score of laboratories, can pack over
a million parts into a cubic foot of space and, at the same
time, greatly increase reliability. Already circuits are available
in quantity, in packages only 0.3 inch in diameter and 0.2 inch
high, to build complete logic and arithmetic sections of computers.
The world's first integrated-circuit electronic control computer
was announced last May. Called "Martac 420," the new computer
was built by the Martin Company at Denver, Colorado. Designed
primarily for ICBM pre-launch checkout and control, it tests
and computes the readiness of all electronics in the missile.
Within the electronic computing system, traditionally made up
of transistors, diodes, and resistors, is logic circuitry comprising
only plug-in integrated circuits. These reduced a conventional
20,000 parts to 5000 and cut over-all size to three-eighths
that of previous construction. One part of this system, a decade
counter with direct-coupled circuitry, is shown in Fig. 2. Here
the integrated-circuit counter is compared to the number of
conventional parts that might have been used.
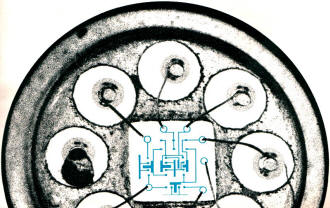
New integrated circuits being worked on in
a score of laboratories promise fantastic size reduction of
much equipment, more reliability, and, eventually, low cost.
Microelectronic development has been encouraged by the needs
of military and space programs. Millions of dollars are now
being spent for research and development. But, as in other areas
of military research, benefits will eventually be passed on
to the public. Military and space programs require smallness,
reliability, and lightness; industry wants low cost and reliability;
the public wants low cost. Microelectronic integrated circuits
offer all these things. Their appearance in all types of equipment,
military and consumer, awaits only the ability to make the wide
variety of circuits needed.
To visualize the future scale of sizes compared to present
and past equipment, the graph of Fig. 3 shows theoretical parts
per cubic foot, ranging from World War II electronic equipment
to the human brain. It is difficult to conceive the ultimate
theoretical smallness, but the human brain is probably close
to this limit, having a density of over 100 billion parts per
cubic foot.
Integrated circuits should perhaps more rightly be called
functional blocks, because each is a single device performing
a complete electronic operation. Such functions might include
one or more stages of amplification, or several switching stages
to perform a computer logic step, such as storing numbers or
counting.

Steps in the manufacture of "Micrologic"
circuitry
Fig. 1 (A) Oxide Growth. Silicon wafer is placed into a furnace
containing oxidizing atmosphere at 1200°C. Oxygen penetrates
crystal surface to form inert, stable silicon dioxide.
Fig. 1 (B) Isolation Masking. Photo-etching technique is then
used to remove surface coating in such a pattern as to produce
separate areas for individual transistors and resistors.
Fig. 1 (C) Isolation Diffusion. Wafers are placed in high-temperature
furnace with gaseous boron. Boron diffuses through the openings
made by lines to produce "p" material.
Fig. 1 (D) Masking and Base Diffusion. Wafer is then photo-etched
again to produce openings for transistor base and for resistors.
Boron is again used at high temperatures to diffuse "p"-type
material into "n"-type silicon, forming base, resistors.
Fig: 1 (E) Emitter Masking and Diffusion. Another photo-etch
is used to locate the emitter area and collector contacts. In
another high-temperature step, phosphorus is deposited and diffused
to produce "n"-type areas employed for the emitter.
Fig. 1 (F) Contact Areas for Interconnections. Next, photo-etching
is used to produce cut-out areas that will be used for interconnection
contacts. The wafers are then placed in a vacuum chamber where
aluminum is evaporated from a hot filament to produce an even
coating of the metal over the surface.
Fig. 1 (G) Metal Interconnections. In a final step the aluminum
layer is masked and etched to form interconnections.
Various Design Techniques
One form of microelectronic circuit in common use today is
made from a single silicon semiconductor crystal, with no external
circuit wiring or components. Wiring is thus reduced to between-circuit
interconnections, which may be printed boards. A typical integrated
functional block is shown in Fig. 4. This is a half-shift register
for computers, containing 9 transistors and 14 resistors all
mounted within an 8-lead transistor-type can.
Intermediate approaches to microelectronics usually comprise
the high-density packing of components, either on or in wafer
substrates, or bunching components together like cordwood with
welded connections.
Another technique, called "thin film," involves the evaporation
of desired metals on the substrate. With suitable masks or stencils,
and the right choice of metals, it is possible to form all of
the passive components (resistors, capacitors, and inductors)
plus interconnecting wires. But it is still necessary to insert
individual transistors and diodes.
The most promising microelectronic concept at present, for
versatility in serving both computer and communication needs
and practical to construct with present scientific knowledge,
seems to be the solid-state integrated functional block.
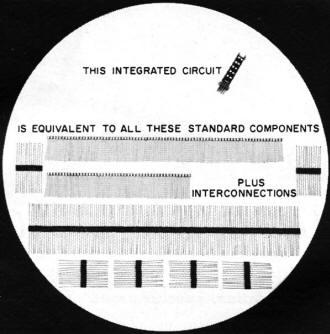
Fig. 2 - Decade counter module and parts
that it replaces.
Maximum Reliability
For military and space electronics the reliability consideration
is probably uppermost. Electronic systems used with weapons,
aircraft, communications, and the control, launching, and tracking
of orbiting or space vehicles, is fantastically complicated
and will be more so in the future. For example, the over-all
reliability of a system equals the product of the reliability
of each of its parts. If the system has three parts, each with
a 90 percent chance of success, then the chance of the system
for successful operation is (0.9 x 0.9 x 0.9 = 0.73) 73 per-cent.
With perhaps billions of components involved in multi-million
dollar space shots and with men's lives at stake, the expectation
of success must be very much greater than that and the problem
is infinitely more complex.
Experience has shown that deep inspection and extensive quality
control are still not adequate to assure high reliability. Costs
are too high and errors can be introduced into the controls
themselves. Therefore, a reduction in the number of individual
components, by making them part of an integrated circuit, is
the only practical way to meet this urgent need. Putting it
another way, the more components, the greater the chance of
failure.
Fabricating the Circuit
To make an integrated functional circuit, which will provide
the active functions of diodes and transistors as well as the
passive components, one begins with a material having semiconductor
potential, such as silicon. The properties necessary for semiconductor
electrical characteristics then require the introduction of
impurities into the silicon crystal. These impurities become
the current carriers, since the pure crystal is not a good conductor.
The atoms of the impurity must be able to combine with the crystal
structure in such a way that an electron is either added or
taken away. The first impurity type is called n and the latter
p, for negative and positive.

Fig. 3 - Packing density for systems and
components.
(After Wallmark and Marcus, "Proceedings of IRE,"
March, 1962)
If p and n characteristics are formed alternately in the
same material, then an electrical barrier is formed. This barrier
will allow current to flow readily when a battery is placed
across it with the negative terminal to the n type and positive
to the p type. But on reversing the battery a high resistance
is presented to current flow.
With present technology, one efficient way of making integrated
circuits with silicon is to introduce a small amount of n impurity
into the entire ingot. Then the n polarity is either counteracted
as required by the addition of p impurity, or the n characteristic
is strengthened by increasing its concentration.
In the manufacture of integrated circuits these impurities
are added to the circuit substrates by diffusion. The units
are placed in a high-vacuum furnace and, at an elevated temperature,
a gas containing the desired impurity is introduced so that
the impurity comes in contact with the substrate and penetrates
its surface. Other methods of adding impurities to semiconductors,
such as alloying, are not suitable for integrated circuits.
The diffusion method is the same as used for the manufacture
of modern high-frequency silicon transistors.
The great advantage of the diffusion method is that many
units can be placed simultaneously in the furnace to make thousands
of devices. With all being exposed to the same conditions, their
uniformity is high, and production yields are high, because
once the process is perfected each individual device tends to
be as good as the whole.
The patterns of diffusion on the substrates are formed by
a photolithographic process similar to that used for etching
plates in printing. Very fine details are possible, limited
only to the degree of control over the precision camera work,
the making of masks for the patterns, and the alignment of the
masks in successive manufacturing steps.

Fig. 4 - "Micrologic" element containing
9 transistors and 14 resistors on base 70 mils square, which
is then inserted into the 8-pin transistor case illustrated
at the right.
Both active and passive components are made into the silicon
substrate material by the same diffusion processes. The methods
employed for the several types of components follows:
1. Resistance is controlled by varying the amount of diffusion
of impurities. More impurity lowers resistance. The photolithography
pattern determines length and width.
2. Capacitors are formed in two ways. In one method, p-n
junctions (barriers) are used, which inherently exhibit capacitance
when reverse biased. In the second method, capacitors are formed
on the surface, with silicon dioxide acting as the dielectric.
3. Inductors of limited value can be formed by diffusing-in
a heavy concentration of an impurity in a spiral pattern. Considerable
development is still required in this area, however.
4. Transistors and diodes are made by successive diffusion
of their parts. These can be made at the same time as the passive
parts, if the impurity concentrations are the same.
"Micrologic" Manufacturing
Typical microelectronic integrated circuits, known as "Micrologic"
by Fairchild Semiconductor, are made by means very similar to
those used for diffused "planar" transistors. This is a device
whose surface is in one plane, with the base and emitter contacts
on the upper surface, and the collector integral with the substrate
material. The previous generation of transistors was the "mesa"
type, which involved etching away of the surface to control
and reduce the size of the base area. True planar transistors
have a hard protecting surface of silicon dioxide, formed by
an oxidizing atmosphere introduced with the diffusions.
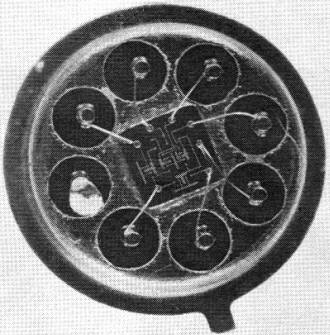
Fig. 5. "Micrologic" element shown mounted
in transistor case (enlarged 6 times).
The "Micrologic" manufacturing steps, from the preparation
of the ultra-pure silicon crystals to final inspection and capping,
are shown in Figs. 1 and 5. Some 60 to 100 circuits are formed
simultaneously in one wafer, and dozens of wafers can be diffused
at one time. This batch processing leads to lower and lower
costs.
The completed unit with leads attached is evaluated for reliability
and electrical performance, by a unique method of testing. Since
individual components cannot be tested, the entire function
is checked for performance over the specified voltage and temperature
ranges .
Of the several approaches to micro-electronics now under
investigation, the integrated circuit seems to have an excellent
chance of long-range and universal use. Reasoning behind this
statement is the integrated circuit advantages of inherently
high reliability, the ingredients required for mass production,
and consequently, low cost.
Other types will have varying degrees of popularity. The
microsized discrete component may lose favor when integrated
circuit development permits all desired functions to be served
by them. The drawbacks of the discrete micro-component approach
are failure to reduce the number of interconnections and high
cost.
Thin-film circuits will logically be used with integrated
circuits to give greater flexibility and a wider range of circuit
values than possible with diffusion alone. But with advanced
integrated circuit technology, the thin-film circuit with individual
transistors and diodes will no longer be required. Admittedly,
however, the transistor was announced only 14 years ago and
we have probably only scratched the surface of the potential
capability, materials, and technologies. Already gallium arsenide
is being investigated for its higher frequency potentiality.
Cryotrons, which switch at zero resistance with very low temperatures,
have been found adaptable to thin-film production. And the field-effect
transistor is coming into its own as a high-impedance amplifier,
competing with vacuum tubes.
The success of the integrated circuit principle, however,
whether in the form described here or in some form yet to be
discovered, seems to be assured, because of the needs of our
modern electronic world. It is not too farfetched to visualize
them in all types of industrial and consumer equipment in the
next five years or so. They will probably be seen in broadcast
and communications equipment, in hi-fi gear, in household electronic
controls, and in small business computers.
Posted July 27, 2015