May 1970 Popular Electronics
Table of Contents
Wax nostalgic about and learn from the history of early electronics. See articles
from
Popular Electronics,
published October 1954 - April 1985. All copyrights are hereby acknowledged.
|
The ambition of amateur hobbyists
has always amazed me. In practically every area of technology, amateurs have contributed
significantly to the effort of pushing back the frontiers of ignorance by developing
new applications, improving existing products, and demonstrating to commercial companies
a need for new features or products. Many of those innovative hobbyists make a living
in the field they choose for off-hours recreation. As a heavy reader of technology
and hobby magazines of all sorts, I know this to be true for musicians, boaters,
pilots, radio operators, gardeners, science experimenters, woodworkers, and many
others. Levels of quality of finished products are awesome in the master class competitions.
The amount of money spent by some for their "hobbies" is shocking. This laser communications
article from the May 1970 Popular Electronics magazine is a prime example
of that. The author uses off-the-shelf components to construct both the transmitter
and receiving components. A quick tally of the most expensive parts - telescopes,
laser module, photocell, power supplies - shows the project would have cost upward
of $400. According to the U.S. Bureau of Labor Statistics'
inflation calculator, $400 in 1970 money is the equivalent of
about $3,056 in 2022 money. That's an expensive "toy."
Popular Electronics Exclusive Developmental Project
Laser Beam
Communicator
By C. Harry Knowles
Audio Modulate Our Low-Cost Laser
Communicating by means of a laser beam is as fresh and new as the tomatoes picked
from your garden tomorrow morning. The mere idea of being able to transmit information
on a beam of coherent laser light suggests all sorts of possibilities for secret,
non-jammable, interference-free communications. And it is possible today!
Communications by laser beam offers several advantages over conventional radio
links. Neither atmospheric lightning nor airborne electrical noise affects laser
communications though they can completely ruin radio communications. On the debit
side, however, laser performance is degraded, over any reasonable distance, by heavy
fog, rain, snow, or terrestrial heat.
Unlike radio, in which the signal is "sprayed" out over a wide area, a laser
beam communications system operates on a line-of-sight basis and the beam is tight
enough to provide excellent privacy. Of course, obstructions cannot be permitted
to interrupt the beam but conventional optical mirrors can be used to bend the light
beam around obstructions if necessary.
PE at the SmithsonianA pair of Popular Electronics laser communicators,
similar to the one described in this article, is scheduled to be shown in operation
at the Laser-10 exhibit at the Smithsonian Institution's National Museum of History
and Technology in Washington, D.C. this spring and summer. Readers living in the
area or visiting Washington will want to see this excellent exhibit, which features
a wide variety of lasers in many unique applications. The POPULAR ELECTRONICS laser
communicators will be set up to carry two-way conversations.
Two approaches to laser communications are described in this article. The first
involves only a simple addition to the basic laser described in POPULAR ELECTRONICS
in December 1969. This system has a range of about 100 ft., and can be used for
experimenting within a room and provides a "breadboard" for use in understanding
modulated laser action. It also makes an excellent science fair project.
The second approach uses a modulation and receiving scheme similar to the first
but it operates through conventional low-cost telescopes to achieve a range of several
miles (depending on atmospheric conditions).
Laser Modulation. The light output of a gas laser such as the
0.5-mw helium-neon type described in our previous article is a function of the current
flowing through the laser tube (see Fig. 1). At very low currents, the laser
becomes unstable and tends to turn itself off. The light output increases reasonably
linear with tube current up to approximately 5 mA. Above that, the light output
drops drastically and tube life is decreased. If the current is centered on the
middle of the linear portion of the curve and varied about that point, the light
output can be made to swing in a linear fashion and very high modulation levels
can be obtained.

Fig. 1 - Light output of the laser is a function of tube
current. Modulation 90% or better can be obtained easily by this modulation method.

Fig. 2 - Negative-resistance characteristic of the laser
shows that a large-value variable resistance is required for stable operation over
the entire operating range.
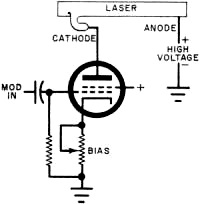
Fig. 3 - The basic amplitude modulator uses a conventional
pentode in series with the laser. The suppressor grid is electrically connected
to the cathode.
The voltage-current curve in Fig. 2 shows that the laser tube has a negative
resistance characteristic (voltage decreases as current increases). Stable, linear
operation thus depends on the use of a ballast resistor. When the tube is operating
at 5 mA, approximately 1100 volts are required. At this point, the negative dynamic
resistance is about 30,000 ohms. As the current is decreased the required voltage
rises until, at about 1 mA, it is approximately 1300 volts. Here the negative resistance
is 80,000 ohms. Therefore, the ballast resistor must have an effective value well
above 80,000 ohms to keep the tube operating.
A basic modulator circuit, using a pentode with a large dynamic resistance, is
shown in Fig. 3. The pentode is in series with the laser tube and forms a simple
amplitude modulator. The dynamic resistance of the pentode is a function of the
applied audio signal on its control grid. A potentiometer in the cathode circuit
of the pentode determines the basic operating resistance of the tube and, hence,
the operating point of the laser. Once the latter point (located on the curve in
Fig. 1) has been set by the bias potentiometer, an audio input to the pentode
causes the laser current to fluctuate about the operating point and the emitted
light is amplitude modulated.
Almost any type of audio driver can be used to generate the input audio signal
to the pentode.
Basic Modulator. The circuit for converting the original laser
project into a light-beam transceiver is shown in Fig. 4. A photograph of the
finished project is shown in Fig. 5. A complete vacuum-tube system is used
simply because a high resistance device is required and the tube that will do the
job is inexpensive and readily available. In addition, the +175 and 6.3-volt sources
required by the pentode can be used elsewhere in the circuit.
The modulator circuit can be divided into two portions. The transmitter (V1)
consists of the pentode modulator driven by the triode half of the tube acting as
a microphone preamplifier. Potentiometer R4 provides modulation level control. The
three gas tubes in series (I1-I3) are 200-volt breakdown lamps which chop off the
high-voltage spikes that trigger the laser. Although the operating plate voltage
of the tube is below its maximum rating, a much higher voltage spike is used to
trigger the laser. The three gas lamps limit this spike to 600 volts. Unlike semiconductors,
a vacuum tube can withstand an overvoltage for a short time. The trigger spike here
lasts only about one millisecond so no damage can be done to the tube. If you can't
locate the gas tubes called for in the Parts List, use any combination of conventional
neon lamps that add up to approximately 600 volts.
The receiving portion of the modulator consists of a three-stage conventional
audio amplifier driven from the output of the solar cell. Unlike a conventional
light-dependent resistor, a solar cell generates a voltage that is a function of
the amount of light striking the photosensitive surface.
Construction. If you built the original laser project, the same
metal chassis may be used. Drill or punch holes for two 9-pin and one 7-pin tube
sockets. These may be located on the top of the chassis, next to the laser tube.
(Be sure to remove the laser tube when doing mechanical work on the chassis.) On
the wall opposite the high-voltage laser power supply, mount the three potentiometers
(R6, bias; R4, modulation level; and R12, receiver volume), the microphone input
jack (J1), and the photocell input jack (J2) (see Fig. 5). Mount power transformer
T2 on the outside of the chassis using the same mounting hardware as were used for
the original 600-volt transformer. (It was T1; now it is T3.)

Fig. 4 - Other than the basic pentode modulator (V1B) circuit,
either vacuum-tube or semiconductor audio amplifiers can be used for the remainder
of the circuit.
Once all the components are installed, wire up the circuit point-to-point (using
terminal strips as required) following the circuit shown in Fig. 4. Of course,
it is not necessary to use vacuum tubes for the microphone amplifier. You can use
the 6AU6 pentode for the laser driver and, for the amplifier, anyone of several
commercially available transistor amplifiers. The author used one of the new RCA
IC kits - the KC4000 microphone preamplifier - in one model and found that it worked
fine. The solid-state receiver consisted of a KC4000 microphone preamplifier for
the photocell preamplifier and a KC4003 1/2-watt audio amplifier to drive the speaker.

Fig. 5 - The prototype was built on the original laser chassis
(December 1969 issue). Any other layout will do as long as the pentode modulator
is as close as possible to the laser.
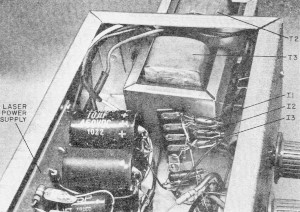
Internal layout of the prototype transceiver (above) showing
the laser power supply mounted on one wall with the rest of the components occupying
the remaining space. The internal arrangement of the telescope electronics (shown
below) shows the modulator tube and its associated components arranged within its
smaller metal enclosure.


The complete telescope system can communicate as far as a 12-inch
target can be clearly seen via the telescope. At night, this target will have to
be illuminated. In good visibility, range can be very great but is dependent on
certain conditions (see text). To assist distant communications, an optional 1-kHz
audio oscillator is used to modulate the transmitter, and both ends must be "juggled"
until the received audio tone is at a maximum. To get around opaque objects, a large-size
front-surface mirror (not a ladies compact mirror) may be used to reflect the laser
beam.
The receiving photocell in this simple light communicator is mounted at one end
of a dark plastic tube. (A cleaned out container of Polaroid print coater works
very well.) If you use a cardboard tube, paint the interior a dull black before
installing the cell. For testing and experimentation, make up a microphone cable
with a phono connector at one end. Use a phone jack to make the connection to the
earphone output of a conventional transistor radio. The radio is silent when the
earphone jack is plugged in and produces a non-tiring audio signal for testing.
Testing. Place the volume, modulation, and bias potentiometers
in their minimum resistance positions. Connect up the speaker, photocell, and radio
and turn on the power. The laser tube will start to blink at a low level until the
modulation pentode warms up. Once the tube is hot, the laser will operate at its
full brightness. A slight increase in the resistance of R6 should cause the laser
beam to dim slightly. This shows that the bias control is operating properly. Now
set the control for full brightness. Increasing the volume control should produce
some hum in the speaker. If conventional room light is allowed to fall on the sensitive
face of the solar cell, it will produce a distinctive hum. This is the reason the
solar cell should be mounted in a dark tube.
Separate the laser and the solar cell by a few feet and aim the beam at the receiver.
Alternatively, aim the laser beam at a mirror so that it is reflected back to the
cell. (The beam must be aimed straight down the cell tube and not at the interior
wall.)
With the laser beam shining on the solar cell at full brightness, turn on the
radio, tune to a station, and plug in the earphone jack. On the laser chassis, turn
up the receiver volume control and note that, as the hand is passed through the
laser beam, a thump is heard in the speaker.
Slightly reduce the bias control to dim the laser a little, and turn up the modulation
control slightly. These two controls interact somewhat so you will have to "juggle"
them for best modulation.
Make sure that the radio volume is turned up sufficiently.
Once the communicator is working, you can experiment with the controls and the
circuit (always retaining the pentode as the laser modulator) to increase your understanding
of laser communications.
Optical Systems. Depending on how you want to use it, the laser
communicator can be set up with anyone of three optical systems. The simplest, which
can be used for point-to-point communications around a room (to a total of 100 ft
round trip), is as described above, without any lenses. To improve the reception
somewhat, a simple lens can be placed in the beam path at the receiver end to reduce
the size of the diverged beam.
The second type of optical system, requires the use of a set of binoculars, one
eyepiece for the transmitter and the other for the receiver. Simple toy telescopes
may also be used. The range for this type of system is a few hundred feet.
For communicating over greater distances, a reasonably high-power telescope is
necessary. Such a telescope, attached to the laser communicator, acts like a high-gain
antenna on a conventional radio system. In both cases the transmitted and received
signals get a boost from the "antenna." And in both cases, the telescope or antenna
is used for both transmitting and receiving through a simple mechanical switching
process.
How far can you transmit using a telescope? It depends on a number of factors,
the most important being beam divergence and atmospheric conditions. As the beam
travels along its path, it tends to enlarge (diverge). This means that, although
the beam leaving the laser is quite small (1 millimeter in the POPULAR ELECTRONICS
laser), it does enlarge considerably - though not as much as a comparable beam of
conventional light. Using a telescope improves this condition considerably.
Atmospheric disturbances of the laser beam cause it to wander.
As the beam of light is projected over a long distance, it may encounter various
forms of air turbulence, such as localized temperature changes. In each of these
turbulences, the density of the air changes and each change in density acts as a
prism as the beam passes through it, changing the beam's direction slightly. The
amount of wander can be as much as several feet per mile. In the still, relatively
even temperature of morning, before the sun has had a chance to warm up the air,
beam wander may be as little as a few inches per mile.
In using a reflector telescope such as that described later in this article,
the beam should be collimated as closely as possible to the distant receiver, allowing
for thermal refractive variations for the time of day and the atmospheric conditions.
If the air is still and of an even temperature, the beam will wander only a few
inches per mile. In this case, also, the beam may be focused so that at the receiver,
the beam diameter has diverged only about one foot per mile. If the atmosphere is
clear, there is little absorption by airborne particulates (smoke, dust, etc.) ;
and the overall result is that about 3 to 5% of the transmitted beam power is obtained
at the receiver. This extremely high efficiency is one of the many attractive features
of laser communications that will help make it the system of the future.
Reflector Telescope Construction. A telescopic system is shown
in Fig. 6. The laser tube is supported by a pair of view-finder ring mounts
attached to the telescope tube. The laser is positioned within the mounts so that
the light-emitting end is almost directly over the telescope eyepiece. (Check your
laser tube to make sure whether the light beam comes out of the anode or the cathode.
Some models are one way; some the other.)
Make up an L-shaped length of heavy bus-bar with the long side about 2 1/2" -
the other about 1" long. Cement (with epoxy) the short end of the bus bar to the
relay armature so that it swings back and forth as the relay is energized and de-energized.
Position the relay about 90° from the telescope eyepiece so that when the long end
of the bus bar is placed through a slot cut in the telescope tube and with the relay
energized (talk position) the end of the bus bar is out of the beam path. With the
relay de-energized (listen position) the wire should be in the beam path. Remove
the telescope eyepiece to watch this.
 
Fig. 6 - Complete telescope communicator showing the use
of semiconductor audio amplifiers. Any neon lamps may be used for I1, I2, or I3
if their breakdown totals up to about 600 volts.
The main chassis for the telescope communicator mounts the relatively heavy power
supplies (except for the laser). all controls, and is connected to the telescope
electronics via a multi-lead flexible cable. Microphone plugs into the rear.
On the solar cell called for in the Parts List of Fig. 4, the black side
is the sensitive area. Cement the shiny side of the cell to the bus bar and then
slide the cell and relay assembly into position. Make sure that the cell switches
cleanly in and out of the beam path as the relay is operated. The two leads from
the solar cell are taken out of the same slit and terminated on a two-lug terminal
strip mounted near the relay.
Mount the empty half of the two-piece electronic chassis on the telescope tube,
just below the two laser mounting rings, drilling mating holes in both chassis and
telescope tube. Use short mounting hardware so as not to interfere with the beam
path. Recheck all mechanical work and tighten the telescope tripod screws.
To keep weight to a minimum, only the modulator pentode and the laser power supply
are mounted in the chassis on the telescope. This is necessary to reduce the possibility
of oscillation in the circuits.
Mount the power supply on the inside of the chassis, using an insulated spacer
(about 1/4") at each corner. Be sure that the high-voltage end is far enough from
the metal to avoid arcing. The seven-pin tube socket for the pentode is mounted
at one end, while a multi-lug terminal strip supports the ends of the wiring. A
1/2" grometted hole should be provided for the incoming cable.
The circuit for the scope-mounted electronics is shown in Fig. 7. Only the
relay, solar cell, and laser are external to the chassis. The circuit above SO1
is mounted at the scope. The lower portion is built in a larger conventional chassis.

View looking into end of telescope shows how the solar cell,
in transmit condition, is out of beam path from laser to diagonal. In the receive
mode, the cell enters the beam path between diagonal and eyepiece. Make sure that
sensitive side of solar cell faces the diagonal.

In the simple transceiver, the solar cell is mounted within a
tube having a dark interior - in this case, it's a clean Polaroid print coater.
Cell is affected by ambient light so that it must be shielded during use. Any method
of mechanical mounting may be used to position the cell correctly.
Once again, either vacuum-tube or semiconductor amplifiers may be used. The latter
save quite a bit of work. Connections between the two chassis are made with multi-lead
cable, with the exception of a small coaxial cable for the solar cell leads. Make
the connections long enough to allow plenty of space between the telescope and the
other chassis. The cables may be taped at intervals to keep them from separating.
When all electronic work is finished, attach the second half of the chassis to
the one on the telescope. The cable should be placed where it will not interfere
with scope operation.
Fully open the ring mount thumb-screws and slide the laser into position as described
above. Tighten the thumb-screws gently to avoid damaging the tube. Attach the plus
side of the high-voltage supply to the laser anode and the negative side to the
cathode.
Make up a phono connector to connect the solar cell leads to J1. Connect the
two leads to the relay.
A small 90° prism is cemented to a plastic block to aim the laser light at
the telescope eyepiece. The plastic block is press fit to the laser end.
The transmit-receive relay is mounted to the telescope tube with the solar cell
and rod passed inside through a hole cut in the telescope tube wall.
Setup. Connect the far end of the multi-lead cable to the main
chassis, along with the solar cell and microphone connectors. (You can substitute
a radio for the microphone for testing.) The push-to-talk button may be temporarily
shorted to keep the solar cell out of the beam path during the following optical
alignment.
Three commercial IC audio kits were used for all stages except the pentode modulator.
Power supplies are mounted under the chassis. Telescope cable termination is on
rear apron.
It is assumed that the telescope optics have been set up as described in the
telescope operating manual.
On the main chassis, set bias control R4, volume control R5, and modulation control
R6 to minimum resistance. Plug in the 117-volt line cord and turn on the power.
The laser tube will blink a few times until V1 warms up. After the laser starts
to glow at full power, allow the entire system to stabilize for a few moments. Adjusting
the bias control should cause the laser glow to diminish a little. Set this control
for maximum laser brilliance.
Place the 90° plastic prism over the protuberance at the laser exit hole and
adjust the prism so that the laser beam is reflected down the telescope eyepiece.
Aim the telescope at a wall and keep adjusting the prism - and if necessary the
position of the laser - until a red circle, with the diagonal mirror shadow centered
in it, is clearly visible on the wall. At this point, the laser has been properly
set up and should not be moved.
If you have to keep looking at the laser beam, a pair of blue sunglasses may
be worn to reduce the red glare.
To test the system, aim the telescope at a distant mirror and reflect the beam
back to a duplicate solar cell that has been connected to the main chassis. You
can also use the second telescope of the communications system if you have built
it at this time.
With the light beam shining on the solar cell, make sure that the radio is playing
at a reasonable volume and turn up the laser volume control R5. If artificial light
falls on the solar cell, a hum will be heard; so for best reception keep the ambient
light dim. Slowly adjust the bias control (R4) until the laser dims a little. Then
bring up slightly the modulation control (R6) until music is heard from the main
chassis speaker. Since R4 and R6 are interlocking in their action, you will have
to adjust them together to get the desired results. If R4 is set for too low a beam
level and R6 is set too high, modulation peaks may extinguish the laser. The automatic
power supply will retrigger the laser, but the controls should be adjusted to prevent
the drop-out. Once clean modulation has been obtained, the radio can be replaced
by the microphone and R6 adjusted for this type of input.
Posted December 14, 2022 (updated from original
post on 9/14/2012)
|