September 1960 Popular Electronics
Table
of Contents
Wax nostalgic about and learn from the history of early electronics. See articles
from
Popular Electronics,
published October 1954 - April 1985. All copyrights are hereby acknowledged.
|
Mechanical meter movements
have been around since the late 1800s. In 1882
Jacques-Arsène
d'Arsonval and Marcel
Deprez developed a meter movement with a stationary permanent magnet and a moving
coil of wire which survives today as the dominant form. Lord Kelvin's (aka
William
Thompson) galvanometer preceded d'Arsonval's by a decade or so, but it relied
on the Earth's magnetic field and needed to be properly oriented to work. d'Arsonval's
movement incorporated a permanent magnet instead to improve sensitivity and convenience.
I'm not sure d'Arsonval gets sole billing on the name - why not the Deprez movement?
This article in Popular Electronics magazine from 1960 is as relevant today
as it was more than half a century ago.
Meters
By Ken Gilmore
The great English scientist, Lord Kelvin, once
said, "When you can measure what you are speaking about and express it in numbers,
you know something about it." Following Lord Kelvin's line of thought, if we are
to know something about electricity and electronic instruments, we must have rugged,
convenient, accurate instruments able to measure a wide variety of voltages, currents,
and resistances. The "meter" is such an instrument.
This most basic of all test instruments has actually not undergone a single change
in fundamental theory or design since 1888. It was in that year - almost 20 years
before the invention of the triode vacuum tube - that Edward Weston developed the
device we now know as the Weston movement.
The Weston Movement
Figure 1(A) shows a single coil of wire suspended between the north and south
poles of a magnet. Figure 1(B) is a cross section of this setup; the arrows indicate
the direction of the magnetic lines of force. When current begins to flow in the
wire, a magnetic field forms around it as shown by the small circles. This field
opposes the field of the permanent magnet so that the coil is forced to rotate as
shown. Since the force generated by one turn is very weak, many turns are used in
practical meters.

Mechanical meter movements
Figure 2 shows such a coil with a needle attached to it; the needle moves along
a calibrated scale as the coil rotates. The distance the needle moves is proportional
to the amount of current flowing. In other words, if 0.5 ma. generates a magnetic
field powerful enough to deflect the needle to half scale, then 1 ma. will cause
full-scale deflection. Figure 3 shows the actual construction of a Weston movement
- the moving coil (A), a magnet and core (B), and the complete movement (C).
Only one aspect of the Weston movement has changed with succeeding years. (See Fig. 4).
Development of better magnetic steels has allowed designers to make a more compact
instrument by putting the magnet inside the moving coil; the iron shell around the
coil completes the magnetic path.Bear in mind that the Weston movement is a d.c.
instrument. If a.c. is applied, the needle will try to follow each reversal of the
current. But since it cannot move fast enough, it remains in one place and vibrates.
But the Weston movement can measure a.c. currents with the addition of a simple
rectifier. Figure 5 shows the basic full-wave circuit commonly used.
This ability of a Weston movement to respond to either a.c. or d.c. current -
with the proper circuitry - makes possible one of the most useful test instruments
in electronics: the multimeter. (See Fig. 6.) By adding a handful of resistors,
rectifiers, and switches to the meter movement, we come up with a versatile instrument
that can measure not only a.c. and d.c. current, but also voltage and resistance.
Measuring Current
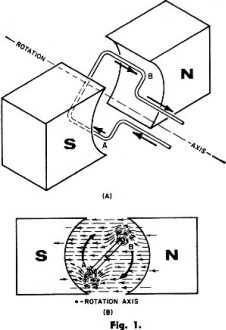
Mechanical meter movement diagram.
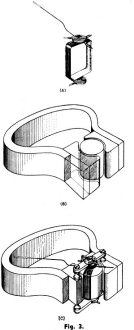
Mechanical meter movement magnet.
Let's suppose we have a meter with a basic 1-ma. movement. This means that if
1 ma. of current flows through the coil, the needle will be deflected to its full-scale
reading. But say we want to measure a current of 2 ma. We can do it by using a "shunt."
To our British friends, this word means a railroad siding. In electronics, it also
means a siding, but one for electrons rather than trains. In Fig. 7, the resistance
of shunt RS1 is equal to the resistance of the meter (Rm).
Thus, half the current (Im) will flow through the meter, the other half
(Is) through the shunt, and the full-scale deflection of the needle represents
2 ma.
Shunts are easily calculated if we know the meter's internal resistance (Rm).
In this case, let's say Rm = 100 ohms. Thus, RS1 in the example
given would also be 100 ohms. If the shunt were 50 ohms (RS2), twice
as much current would flow through the shunt as through the meter. The meter would
conduct only one-third the total current, and its full scale deflection would represent
3 ma. If the shunt were approximately 11 ohms (RS3), nine times as much
current would flow through the shunt as through the meter, or, conversely, one tenth
the total would flow through the meter, and the full-scale sensitivity would be
10 ma.
Modern multimeters have a number of different shunts which can be switched into
the circuit to give different current ranges. One typical meter on the market, for
example, has scales of 1.5 ma., 15 ma., 150 ma., 500 ma., and 15 amperes. Incidentally,
the shunting circuits work the same way in both a.c. and d.c. circuits; the only
difference is that a rectifier must be in the circuit for the meter to read a.c.
Measuring Voltage

Mechanical meter movement coil.
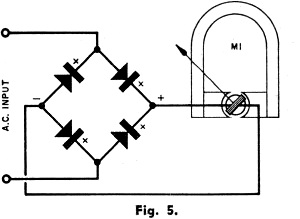
Mechanical meter movement diode bridge for AC input.

Typical multimeter with mechanical movement.

Mechanical meter movement range selection schematic.
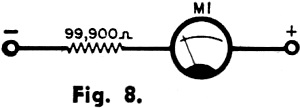
Schematic for meter movement.
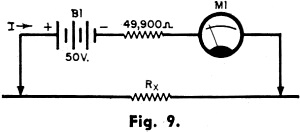
Measuring external resistance.

Printed circuit meter movement.

Meter movement product applications.
So far, we have considered only current measurements. But a meter can be connected
to measure voltage as well. Let's take that same basic 1-ma., 100-ohm meter movement
again and make a voltmeter out of it. By using Ohm's law, we can find the voltage
which must be applied across the meter terminals to make a 1-ma. flow: E = IR; E
= .001 x 100; E = .1 volt.
If more than .1 volt appears across the terminals, more than 1 ma. will flow
through the meter and damage or destroy it. But suppose we want to measure 100 volts.
Again, we apply Ohm's law to find out what resistance the meter would need for only
1 ma. to flow if 100 volts were applied: R = E/I; R = 100/.001; R = 100,000 ohms.
Since the basic movement is only 100 ohms, we simply add the 99,900 ohms to make
up the total of 100,000 in series with the movement as shown in Fig. 8. With
various resistances switched into the circuit, one meter can measure a wide range
of voltages. One typical commercial meter, for example, has ranges of 1.5 volts,
5 volts, 150 volts, 500 volts, 1500 volts, and 5000 volts. Again, both a.c. and
d.c. voltage ranges can be measured by switching in the rectifier for alternating
current.
Measuring Resistance
A basic meter movement can even be made to measure resistance, but this requires
an additional circuit component - a battery. Let's take our 1-ma., 100-ohm meter
movement and connect a 50-volt battery in series with one of the leads, as shown
in Fig. 9. Again, Ohm's law comes into the picture. We know we have 50 volts
in the circuit, and the meter movement must not conduct more than 1 ma., so we can
calculate the minimum resistance that must be included in the circuit to limit the
current to a 1-ma. value: R=E/I; R=50/.001; R=50,000 ohms.
Since the meter already has an internal resistance of 100 ohms, we need add only
the other 49,900 in series (R1). Now if we short the two test leads together,
1 ma. of current will flow and the meter will read full scale. Any additional resistance
introduced into the circuit will cause the meter to read somewhere between full
scale and zero. A relatively low resistance (Rx) between the test leads,
for example, would perhaps make the meter read nine-tenths full scale; a larger
resistance would make it read half scale; with an infinite resistance, no current
at all would flow.
Thus, we see that zero ohms appears on the right end of the scale, or opposite
from the zero of the volt and ampere scales. Because of the inherent characteristics
of the ohmmeter, the needle becomes more and more inaccurate as it approaches the
left side of the scale. Therefore, technicians usually try to take resistance readings
as near the center of the scale as possible, to obtain the most accurate readings.
They do this by switching to various ranges, which, in turn, means switching batteries
of different voltages into the circuit. In practice, also, a small variable resistor
is usually included in the ohmmeter circuit to compensate for variations in battery
strength. The knob controlling this resistor is usually labeled "Ohms Adjust" on
the front panel of the multimeter.
Other Uses
The basic Weston movement can be incorporated into still other kinds of circuits
containing vacuum tubes. These instruments have certain advantages over the conventional
multimeter just described, and are particularly useful for some types of work. (See
Test Instruments: The Vacuum-Tube Voltmeter; April, May, July, 1959, P.E.)
Except for certain kinds of vacuum-tube voltmeters, all multimeters have one
great shortcoming: they can measure a.c. voltages at relatively low frequencies
only - up to about 20,000 cps. But radio and television stations and other branches
of communications must measure r.f. voltages and currents up to hundreds of megacycles.
This is done with the help of the thermoelement - two tiny chunks of metal, frequently
constantan and platinum, clamped together. When the metals are heated, they generate
a small voltage across the junction between them. Thus, a circuit can be designed
so that r.f. voltage flowing through a separate conductor will heat the junction,
which then generates a voltage proportional to the amount of heating. This current
is measured by a Weston movement, calibrated in terms of r.f. current.
Designers, using the Weston movement as the basic indicator, have come up with
an astonishing bag of tricks over the years. With the addition of a light-sensitive
selenium disc, for example, as shown in Fig. 10, the device becomes a commercial
photographic exposure meter - the brighter the light on the disc, the more current
generated. A small generator, on the other hand, transforms the meter into a tachometer
for measuring rpm. In another application, two meters in one case can be connected
to the electronic receivers in an aircraft instrument landing system and arranged
so that they show the pilot whether he is on or off course (Fig. 11). Such
meter applications are virtually endless.
New Developments.
Although the basic Weston movement has not changed in principle or basic design
for 72 years, there are many striking new developments in meters. One of the newest
is a printed-circuit meter recently introduced by the Parker Instrument Division
of Interlab, Inc. As shown Fig. 12 the meter's coil is printed on a thin disc
and mounted parallel to a ring magnet. When current flows through the printed-circuit
coil, a magnetic field is created which reacts with the field of the magnet, and
makes the disc rotate. A soft iron shell (not shown) encloses the magnet and disc,
furnishing a return path for the magnetic lines of force.
The entire printed-circuit meter is only 1/2-inch thick. And since it weighs
only a fraction as much as conventional meters of similar sensitivity and range,
it will undoubtedly find widespread use where size and weight are important - in
airborne equipment, for example. Another important advantage of the new movement
is its ability to handle overloads that would instantly burn out the relatively
delicate Weston movement. The manufacturer claims that an overload of 1000 to 5000%
will not damage these movements.
Another relatively new development is the meter which triggers a relay. Here,
the indicating needle is fitted with a contact. A matching contact is fastened to
an arm which is adjustable from the front panel. When the current in the circuit
under measurement causes the needle to deflect to where the adjustable arm has been
pre-set, the two contacts come together and set off a sensitive relay which can
then be used to control some other circuit. By far the most sensitive relays available,
these instruments can be made to operate on as little as one or two microamperes.
Units of this type, by the way, can be used in any kind of control circuit- battery
charging, tube overload protection, etc. - anywhere fast, accurate control is needed.
But developments such as these only scratch the surface. As the science of electronics
advances into new realms, scientists and engineers are constantly finding new ways
to make the basic meter - oldest of all electronic test instruments - more and more
useful.
Posted January 5, 2023 (updated from original
post on 3/27/2013)
|