September 1971 Popular Electronics
Table of Contents
Wax nostalgic about and learn from the history of early electronics. See articles
from
Popular Electronics,
published October 1954 - April 1985. All copyrights are hereby acknowledged.
|
Here is the second of a two-part article on operational
amplifiers (opamps).
Part 1 appeared in the August 1971 issue of Popular Electronics, which
I have and will post soon (it still needs to be OCR'ed). Fortunately, you don't need
it to find Part 2 useful. Barely half a decade had passed since Bob Widlar introduced
his μA709 integrated circuit operational amplifier. Clunky attempts
at vacuum tube operational amplifiers were introduced in the 1950's, but they were not
overly popular. Early bipolar junction transistor opamps offered a significant
reduction in size, weight, and power consumption over tubes, but did not have as high
of an input impedance, had a lower gain-bandwidth product, and handled much lower
power levels, which relegated their use to IF and baseband circuits. Improvements
came rapidly, however. JFET and the MOSFET implementation soon raised input
impedances into the megohm levels, but it would be a couple decades before frequency
and power levels saw a significant increase.
The Operational Amplifier -What It Is & How It Works

Basic Applications for This Very Versatile IC Device
In the first part of this article, we discussed the "perfect amplifier" and its characteristics.
However, there is no such thing as. a perfect amplifier and we must work with things
that exist in the real world. So, how about applications for the real operational amplifier?
Figure 1 shows the characteristics of one typical low-cost op amp (Texas Instruments
SN72709N), which is a member of the famous 709 family.
This device has an open loop gain of 50,000, an input resistance of 250,000 ohms,
an open loop output impedance of 150 ohms, and one microampere input offset current with
two millivolts offset voltage at the output. These are typical specifications for most
709 op amps, regardless of manufacturer.
The best way to experiment with an integrated circuit of any kind without damaging
it in soldering and desoldering is to make up a breadboard similar to that shown in Fig.
2. Suitable solder terminals are mounted on a piece of plastic and the IC is attached
to the board with adhesive with its leads up. Each pin of the IC is then connected to
one of the terminals. Each terminal is identified as to pin number or function, and all
external components and circuits are hooked up to the appropriate terminals.

Fig. 1 - Characteristics of a typical operational amplifier. This
one has a gain of 50,000, an input impedance of 250,000 ohms, and a 150-ohm output.

Fig. 2 - This simple breadboard approach may be used to connect up
an op amp. The layout below shows a circuit fully wired.
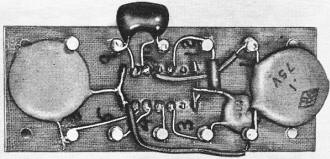
Fig. 3 - If you mount a dual-in-line socket on the breadboard, it
can be r used for both in-line and round IC's (top & bottom views).

Another approach is shown in Fig. 3. Here, a 14-pin dual in-line socket is mounted
on a board, with a suitable number of terminals around the edge. The circuit can then
be built up between the socket leads and the perimeter terminals. Figure 3 also shows
how a round TO-99 case can be inserted in the socket, with the pins properly mated.
Typical Applications. Although only a few circuits will be described
here, they are basic to all of the many variations that are found in this and other publications.
Note that, although some of the circuits shown here do not have compensation, it is always
necessary to compensate a 709. This is not true, however, of some other op amps so the
specifications should always be checked.
The two dc voltmeters shown in Fig. 4 illustrate some interesting points. In both
circuits, the 5000-ohm output resistors can be changed to affect the basic circuit sensitivity.
For example, making this resistor 1000 ohms gives both voltmeters full-scale ranges from
0.1 to 100 volts. Circuit A would then have an input sensitivity of 100,000 ohms per
volt, but circuit B would retain its original 10-megohm input. Circuit B also has a null
balance circuit since the typical offset of a 709, multiplied by the gain of 100, would
produce a significant zero offset on the meter. In this case, with the input shorted,
the null offset potentiometer is adjusted to obtain a zero on the meter. Such a voltmeter
would be ideal not only for solid-state testing (since it has the necessary low-voltage
scale), but also for vacuum-tube circuits where the dc voltage could reach 500.
A very linear ac voltmeter is shown in Fig. 5. In this circuit, diode nonlinearity
is minimized by the high gain of the amplifier. Sensitivity is the same as that of the
meter: that is, 1000 ohms per volt for a 1mA meter. A higher input impedance can be attained
by using an op amp buffer in front of this circuit.
The current-to-voltage transducer shown in Fig. 6 makes use of the current sensitivity
of the op amp to measure very small currents. As shown, the circuit indicates 1 volt
per microampere and is capable of 0.1-microampere sensitivity. Resistance values for
R1 can be between 100,000 and 10,000,000 ohms to provide outputs from 10 μA per volt
to 0.1 μA per volt.
The circuit shown in Fig. 7 is an example of just how far you can go in creating an
ultra-high input impedance with an op amp. Developed by NASA, the circuit has an input
impedance of several hundred megohms with an input capacitance of less than 1 picofarad.
The high impedance is obtained by positive feedback through C1. The input capacitance
plus the capacitance to ground can be cancelled by adding feedback capacitor C2 and properly
adjusting R1.
The low-frequency response is determined primarily by C1, for which an electrolytic
capacitor may be used. High-frequency response is limited by the op amp. With a square
wave applied to the input, potentiometer R1 is adjusted to obtain a square wave on the
output (similar to making a scope attenuator adjustment). The circuit was designed to
amplify a 5-μs pulse coupled through a 1-pF capacitor. The slew rate is approximately
0.5 volt per microsecond.
Two gain-control, or variable-attenuator, stages are shown in Fig. 8. Note that two
different input resistances are shown - one very high, the other low - and that the gain
of either stage can be varied by changing the feedback circuit. A word of caution: when
the feedback potentiometers are at their minimums, the effective load on the amplifier
is 5000 ohms. Be sure that the feedback resistors do not "use up" all the available output
current.

Fig. 4 - A pair of dc voltmeters using an op amp. The circuit at (A)
is 20,000 ohms per volt, while that at (B) is 10 megohms per volt. Text discusses design
changes to improve this simple design. In both cases, the meter has a conventional 0-1-mA
movement.
An interesting use of the op amp is in frequency-selective networks. With conventional
discrete semiconductor circuits, it is usually necessary to use large inductors to perform
this operation at low audio frequencies. In the circuit shown in Fig. 9, a twin-T filter
(which has a resonance similar to its LC counterpart), is used in the feedback circuit.
Figure 9 shows the method of calculating the element values for any frequency. Unfortunately,
the Q of a twin-T filter is rather small - on the order of 0.25; but, when combined with
the gain of the op amp, the Q is a reasonable value. Using an amplifier with a gain of
10, the Q is 2.5; and with a gain of 40, the Q is 10. Thus an op amp, with a few passive
components, can be used to simulate a bulky, expensive inductor; and it has the advantages
of a center frequency and Q that are easily controlled over a wide frequency range.
Another audio filter, this one generating a notch at the selected frequency and having
a variable Q, is shown in Fig. 10. The input to the positive terminal of the op amp is
combined with feedback through the bridge-T network. The other input is variable. When
the signal levels at both inputs are equal, there is no output from the amplifier. System
gain is still R2/R1. By adjusting the "SET" control, a small notch at the filter frequency
is obtained. As the "Q ADJUST" control is brought near the filter end, the feedback increases,
controlling the Q of the circuit. The frequency is determined by the values of the filter
capacitors and the setting of the ganged potentiometers.
Performance Limitations. Input limitations are applicable primarily
to follower configurations, provided, of course, that input overloads are avoided. The
summing junction of an inverter remains at ground except when fast voltage spikes or
extremely high voltages are applied. In the first case, feedback is too slow to protect
the summing junction; while in the second case, the output stage saturates and is unable
to divert the input current. In followers, the summing junction moves in step with the
input voltage so that, in some circuits, the input must be restricted to 15 volts. In
Fig. 4B, for example, the divider restricts the summing junction excursions until the
output stage saturates. The amplifier has a gain of 100 (101 if the resistor values are
exact), and an input in excess of 0.1 volt would saturate the amplifier at an output
over 10 volts. If a 10-volt input were allowed, the summing junction would be driven
so high that the input transistors in the op amp would probably be destroyed.

Fig. 5 - Typical ac voltmeter basic circuit using an op amp. Although
the input impedance is only 1000 ohms per volt, by adding an op amp buffer in front,
impedance can be raised.

Fig. 6 - This current-to-voltage circuit indicates 1 volt per microampere
and is capable of at least 0.1-microampere sensitivity.

Fig. 7 - This circuit, developed by NASA, has an input impedance of
several hundred megohms with an input capacitance of less than 1 pF.

Fig. 8 - A pair of variable attenuator circuits. The upper one has
an input resistance of 1000 ohms, while the bottom circuit has a 50-megohm input. Gain
is very similar.
Performance limitations having to do with offset voltage and current are largely inconvenience
factors. External null circuits balance out offsets over a small range of ambient temperatures.
Offset effects (as well as open loop gain and input resistance) vary with ambient temperature,
so circuits that must operate in changing temperatures should be designed around amplifiers
with low offset. Op amp circuits with low values of R1 and R2 are not bothered by offset
currents, while circuits with low closed loop gain suffer little from offset voltage.
For special applications, where extreme accuracy and/or stability is needed, it is important
to consider not only the open loop characteristics of the amplifier, but also the accuracy
and temperature stability of the external components.

Fig. 9 - A frequency selective network in the feedback loop of an
op amp can simulate an LC circuit having a high Q at audio frequencies. Both Q and center
frequency can easily be changed by varying the RC values.

Fig. 10 - This audio filter permits changing both Q and center frequency
via pair of controls.
In choosing an amplifier for a given application, the manufacturer's specification
sheets should always be consulted. Unfortunately, these sheets often contain an amazing
amount of information - which may be confusing to the uninitiated. Figure 11, for instance,
shows part of the information given on the Fairchild μA748 op amp. Note the two columns
headed "709" which have been added to the illustration for comparison purposes. Some
of the performance figures have been underlined. These are "worst case" conditions and
should be used in circuit design. Also note that some specifications are accompanied
by "conditions" (such as a specified load resistor). When comparing amplifiers, these
conditions must always be identical. All specifications are always for the open loop
configuration unless otherwise noted on the sheets.
By now, you should have a pretty good idea what an operational amplifier is and how
it is used. The next step is to keep your eyes open as you review the technical literature
and be aware of the wide variety of op amp circuits available. Then put them to good
use.

Fairchild Linear Integrated Circuits μA748
General Description - The μA748 is a high performance monolithic
operational amplifier constructed on a single silicon chip, using the Fairchild Planar*
epitaxial process. It is intended for a wide range of analog applications where
tailoring
of frequency characteristics is desirable. High common mode voltage range and absence
of "latch-up" make the μA748 ideal for use as a voltage follower. The high gain and
wide range of operating voltages provide superior performance in integrator, summing
amplifier, and general feedback applications. The μA748 is short-circuit protected
and has the same pin configuration as the popular μA741 operational amplifier. Unity
gain frequency compensation is achieved by means of a single 30 pf capacitor.
Fig. 11. This is a portion of the specifications sheet on the
748 op amp with the "worst case" parameters underlined. The specs of a typical 709 have
been added for comparison purposes.
Posted October 10, 2018
|