September 1974 Popular Electronics
Table of Contents
Wax nostalgic about and learn from the history of early electronics. See articles
from
Popular Electronics,
published October 1954 - April 1985. All copyrights are hereby acknowledged.
|
Single-sideband
(SSB) modulation was a big deal when it was first introduced at a time when the
radio spectrum was getting pretty crowded - particularly in the commercial
broadcast bands. It allowed more channels / stations to be packed into available
space, which was obviously a good thing, but the other main benefit of SSB is
the transmission efficiency - especially when a suppressed carrier format is
used. At really high powers, electronic components, antennas, cabling, and
facilities are very expensive, so being able to broadcast a signal over a given
distance at a lower power represents a cost savings. As the old saying goes,
though, "there is no free lunch." Modulators in transmitters require more
circuitry and much be kept in alignment in order to properly operate in single
sideband mode. Receivers, too, are more complex as the requirement for carrier
recovery (for suppressed carrier SSB) and other accommodations are needed. This
article from a 1974 issue of Popular Electronics magazine does a great
job of introducing the uninitiated into the world of single sideband.
Methods of SSB signal generation, transmission and reception
By James E. Trulove
Single-Sideband, or SSB, modulation has begun to supplant conventional amplitude
modulation (AM) in electronics communication. Many military and commercial systems
have made the switch. So, too, have a great many amateur radio operators. More recently,
Citizens Banders have joined the ranks of the SSB communicators.
SSB modulation has distinct advantages in communications. One of the most important
is its inherent conservation of the crowded frequency spectrum. When compared to
conventional AM, SSB requires only half the spectrum space, in effect doubling the
number of channels i n a given portion of the frequency band. A good example of
this is the 27-MHz CB band where only 23 channels are available with AM, while 46
channels of SSB fit into the same band.
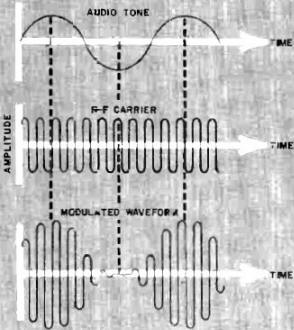
Fig. 1 - In amplitude modulation, audio tone (top) combines with
r-f carrier (center) to produce waveform at bottom whose envelope varies in amplitude
and frequency with the audio tone.
SSB is also much more efficient than AM. In AM, most of the power is concentrated
in the r-f carrier. By contrast, SSB suppresses the carrier and concentrates most
of the power in the information-bearing sideband signal. This, coupled with greater
immunity to selective fading, gives SSB communication much greater "talk power"
than is possible with conventional modulation techniques.
The Basis of SSB
To transmit a signal over a given distance, information is imposed on a r-f wave.
The r-f wave serves as a "carrier" - hence its name - for the lower-frequency information.
Successful communication results from proper utilization of the frequency-dependent
properties of the carrier, as well as efficient use of the transmitted energy.
The information can be imposed on the r-f wave by modifying, or modulating, the
carrier as the result of varying the carrier's frequency or amplitude (or both).
Frequency modulation (FM) and amplitude modulation (AM) and simultaneous amplitude
and frequency modulation (AM/FM) can take a wide variety of forms.
AM in its basic form is perhaps the easiest to produce. To impress an audio-frequency
(a-f) modulation on a r-f carrier, the carrier is made to vary in amplitude according
to the instantaneous amplitude of the a-f signal by a process known as "mixing."
In Fig. 1, the audio and carrier waveforms are combined to produce the modulated
r-f wave shown at the bottom. Notice that the relative peak-to-peak amplitude of
the modulated wave varies in accordance with the relative amplitude of the modulating
audio.
Mathematically, the amplitude-modulated signal contains several discrete frequencies
for any given carrier and audio inputs. As shown in Fig. 2A, the output consists
primarily of the original and carrier signals, plus two additional mixing products
whose frequencies are the sum and difference of the a-f and r-f signals. Called
"side tones," these two frequencies are equally spaced on either side of the r-f
carrier's frequency. (There are many other mixing products, including harmonics
of the inputs and outputs and their mixing products, but these are almost negligible
and need not be considered in this discussion.)
When the audio input is of varying frequency and amplitude, as is the case with
voice, the side tones vary in strict accordance, producing what may be termed "sidebands"
on either side of the carrier. The sidebands form the characteristic "envelope"
of the modulated waveform. These sidebands contain all the original audio information
and are essentially mirror images of each other. With 100-percent modulation, the
carrier contains twice as much power as the total in both sidebands, yet the carrier
conveys no usable information. Also, since the sidebands are mirror images of each
other, only one is needed for effective communication. This means that what remaining
power there is must be divided between the two sidebands. Hence, under the very
best of modulating conditions in AM, less than 25 percent of the available power
goes into the information signal.

Fig. 2 - Frequency components of AM and SSB signals are shown
in (A) and (B), respectively.
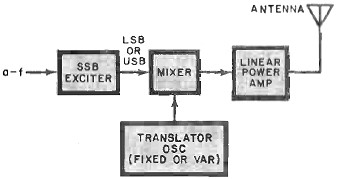
Fig. 3 - Basic block diagram of SSB transmitter.
In SSB communications, one sideband and virtually all of the carrier are eliminated.
After all, the audio signal already has both frequency and intensity. The frequency
information is transmitted as the difference between the carrier reference and side
tone frequencies. The intensity information is characterized by the amplitude of
the side tone. So, one sideband of an amplitude-modulated wave can carry the audio
signal if the reference frequency of the original carrier is available. The suppression
of the carrier and elimination of one sideband permits all of the available power
to be concentrated in the information-bearing signal (see Fig. 2B), effectively
multiplying power efficiency while halving the bandwidth required.
An interesting aspect of the SSB signal is that it disappears when there is no
modulation. (In conventional AM, the carrier is present regardless of whether or
not it is modulated. In other words, the carrier has accompanying sidebands only
when modulated.) In SSB, filtering out all but one sideband causes the sideband
to disappear when modulation ceases.
The SSB Exciter
Unlike conventional AM, the SSB signal is usually generated at a low power level
in separate transmitter stage known as an "exciter." SSB signal generation is easier
to accomplish at a fixed frequency. The output of the exciter is translated in a
mixer to the desired transmit frequency and is then amplified to the desired power
level. (See Fig. 3.)
The balanced modulator, a type of mixer that suppresses the carrier at its output,
is basic to the SSB exciter. The basic types of balanced modulators shown in Fig..4
include shunt, series, and ring (or double-balanced) types. The shunt and series
types may contain two or four diodes, with the latter versions often referred to
as "bridge" or "quad" modulators.
The operation of the balanced modulator can be visualized as an audio signal
being switched on and off at the rate (frequency) of the r-f carrier. If the modulator
is in balance, the carrier is cancelled at the output and only the two sidebands
remain. Balancing of the modulator is achieved by close matching of the diodes and
by trimming. In a properly designed and balanced circuit, carrier suppression can
be as much as 50 dB. The ring modulator provides good carrier suppression with higher
output voltages and lower unwanted mixing products than both the shunt and series
modulators.
After the double-sideband (DSB) suppressed-carrier signal is generated in the
balanced modulator, one of the sidebands must be eliminated by filtering,. This
is no easy task because the two sidebands are very close together in frequency.
If the lowest modulating frequency is 300 Hz, the sidebands will be only 600 Hz
apart. Hence, filtering must be accomplished by a very high-Q filter with a narrow
passband and steep side skirts. A relative comparison between an ideal filter, a
multiple-pole crystal or ceramic lattice filter, and an ordinary LC filter is shown
in Fig. 5A. From the evidence of the curves, it is obvious why a crystal or ceramic
filter is the usual choice in SSB.

Fig. 4 - In SSB, carrier and r-f signals are mixed in balanced
modulators. Outputs have suppressed carriers.

Fig. 5 - Relative comparison between ideal, crystal or ceramic
and LC filters shown at (A). (B) shows elements of SSB exciter.
Since the filter is designed for a fixed frequency, some method must be used
to permit selection between the upper sideband (USB) and lower sideband (LSB). Two
expensive filters can be used, but it is simpler and more economical to switch the
carrier frequency input to the balanced modulator. For example, if the filter's
output is the USB signal, the carrier oscillator can simply be raised by a fixed
amount (usually 3 kHz) so that the LSB signal is at the filter's frequency. The
carrier oscillator is usually crystal controlled. So, the change from USB to LSB
and vice versa can be easily accomplished by switching crystals as shown in Fig.
5B.
The "phasing" exciter is another type sometimes encountered in SSB. It has two
balanced modulators and two phase-shift networks, one for audio and the other for
the carrier. The modulator outputs are combined in such a manner that one sideband
is reinforced while the other is cancelled. This scheme requires critical phasing
for each frequency if no frequency translator is provided. Another disadvantage
is that circuit complexity is considerably greater than with the filter method.
Frequency Conversion
Since filter requirements force the exciter to operate at a fixed frequency,
a converter must be used to translate the exciter's output to the operating frequency.
The converter consists of a mixer with a tuned output and an oscillator that can
be either fixed or variable, depending on the requirements. The mixer produces the
sum and difference products of the output of the exciter and the converter oscillator.
The tuned mixer output passes the mixer product at the transmitter frequency.
To illustrate this process, refer to Fig. 6. Here, a 9-MHz SSB signal, mixed
with a 19-MHz crystal oscillator frequency, produces sum and difference products
at 10 and 28 MHz. The tuned output allows only the 28-MHz signal to pass. The 28-MHz
signal has acquired the SSB-modulation from the 9-MHz exciter output. The signal
can now be amplified and transmitted.
The conversion from 9 to 28 MHz is referred to as "up-conversion." Similarly,
"down-conversion" can be used to convert from 9 MHz to, say, 7 MHz. If a band of
frequencies, such as from 28.0, to 28.5 MHz, must be covered, switching crystals
from 19.0 to 19.5 MHZ could be used in the converter. However, this can become cumbersome
if many frequencies are required. Frequently, a variable-frequency oscillator (vfo)
is substituted in the converter to allow continuous coverage of a given frequency
increment. A vfo with a 500-kHz range from 19.0 to 19.5 MHz would do the job, but
it must be very stable to obviate any drift in frequency.
The converter provides two choices for the oscillator frequency that will produce
the desired output. In the above example, a 37-MHz oscillator would produce primary
mixing products of 28 and 46 MHz. The choice between the two oscillator frequencies
is based on a number of factors, including oscillator stability, mixer efficiency,
crystal stability, and cost. If the difference product is selected, as in the 37-MHz
case, an inversion of the sideband occurs. This means that, if the 9-MHz exciter
output was USB, the converter, output produced by subtracting 9. MHz from 37 MHz
would be the 28-MHz LSB. This phenomenon does not occur when the sum product is
selected.
Linear Amplifiers
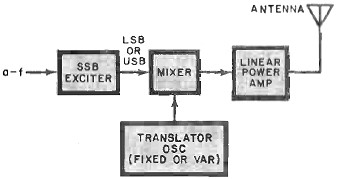
Fig. 6 - Typical converter stage translates exciter output to
desired transmit frequency.

Fig. 7 - Distortion products created by r.f. amplifier nonlinearities
create "splatter" on adjacent frequencies.
Once the SSB signal at the proper frequency is generated, it must be amplified
to the desired output power level. Both AM and SSB signals can be severely distorted
by any nonlinearities in the driver or power amplifiers. In an AM transmitter, this
problem is usually avoided by modulating the carrier in the final r-f stage. This
"high-level" modulation cannot be used in an SSB transmitter where a low-level exciter
signal must be greatly amplified. For this reason, it is important that amplifier
stages be extremely linear to limit distortion to an absolute minimum.
The distortion products created by amplifier nonlinearities can appear in and
around the SSB signal at appreciable power levels. Distortion products are produced
by means similar to those in the mixer. To illustrate, if a two-tone 500- and 900-Hz
test signal were applied to the audio input using a 1000.0-kHz USB signal,
the modulation would appear at 1000.5 and 1000.9 kHz. In Fig. 7,. the lower-order
distortion products resulting from harmonic mixing are shown. Notice that some of
these products are within the normal bandpass and others are adjacent to it. These
spurious outputs can cause significant interference to adjacent channels and, if
distortion is very bad, even frequencies far removed.
There is another common source of distortion that can be confused with power
amplifier nonlinearity. This is distortion at the audio input due to either excessive
clipping or compression in the audio stages or overmodulation.
The two-tone signal can also be used for determining the power contained in an
SSB signal. The waveform of a two-tone SSB signal is shown in Fig. 9.The signal
is rated in peak-envelope power (pep) developed by two tones of equal amplitude.
Pep can be calculated by squaring the rms value of the peak, envelope voltage Ep
and dividing the result, by load resistance R (pep = Ep2/R).
In the two-tone case, the pep is twice the average power dissipated in the load.
Receiving SSB
The SSB receiver is similar to any other superheterodyne receiver (see Fig. 9).
Nonlinearities in the r-f or i-f amplifiers will cause distortion in the SSB receiver
just as they will in the AM receiver. Distortion due to overloading the amplifier
is reduced by the automatic gain control (agc).
The agc must be designed differently for SSB reception. Unlike AM where the carrier
is present even when there is no modulation, the SSB signal disappears completely
in the absence of modulation. For this reason, the agc must reduce amplifier gain
quickly when it first senses a strong SSB signal and increase the gain very slowly
when the signal disappears. This fast-attack, slow-release response compensates
for short pauses in conversation. In some receivers, the sustaining time of the
agc can be adjusted as desired.

Fig. 8 - Diagram shows how two-tone SSB envelope can be used
to calculate pep of SSB signal.
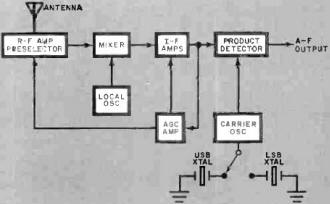
Fig. 9 - Basic SSB receiver is similar to other superhets. Note
crystal switch to select sideband.
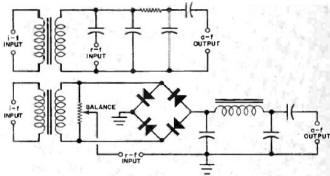
Fig. 10 - Two examples of types of product detectors that are
used in SSB signal demodulation.
The r-f amplifier, mixer, and local oscillator are similar to the circuits found
in AM receivers. The i-f amplifier, however, can be much narrower in bandpass since
the SSB signal is less than half the bandwidth of an AM signal. Reducing i-f bandwidth
has the added advantage of reducing the total noise power presented to the detector.
Frequently, the local oscillator frequency is selected so that the high-Q mechanical
or crystal filter in the SSB exciter can also serve as the i-f filter.
SSB demodulation is radically different from AM detection. As mentioned earlier,
the SSB signal varies in frequency from a reference, depending on the modulating
signal frequency. The reference was originally supplied by the r-f carrier. That
reference carrier must again be simulated to provide something with which to compare
the SSB signal frequency. Thus, the receiver must inject a simulated carrier into
the detector by means of a carrier-reinsertion oscillator or beat-frequency oscillator
(bfo). The bfo is usually crystal controlled to maintain a high order of stability.
The proper bfo frequency depends on whether the signal to be received is USB
or LSB. In the case of USB, the bfo frequency must be lower than the signal frequency,
while for LSB it must be higher than the signal frequency. Crystal switching changes
the bfo from the lower edge of the i-f passband to the upper edge to receive USB
or LSB.
The actual process of detection can occur in an AM-type integrating detector
after the bfo signal is injected. But much higher quality detection can be obtained
with a "product detector." (Examples of product detectors are shown in Fig. 10.)
The quality of the received audio depends to a large extent on how dose the reinserted
carrier is to the frequency of the original carrier. To allow for same drift or
misalignment between the transmitted signal and the receiver's local oscillator
and bfo, a means of adjusting one or both oscillators must be included. A trimmer
for either oscillator might go under various names, the two most popular being "fine
tuning control" and, in the case of CB radio, "clarifier." In some sophisticated
receivers, this function is accomplished by an automatic-frequency control, similar
in function to the afc circuit used in FM receivers. Without the aforementioned
control, speech would be unintelligible.
In Conclusion
The use of SSB modulation offers distinct advantages for the modern communicator.
Especially in the high-frequency bands, SSB conserves valuable spectrum space. Where
the total output power (or input power to the transmitter's final amplifier) is
limited by government regulation, SSB packs all the power into one no-nonsense information
signal to provide greater communicating range and reliability. If maximum power
is limited by tubes or transistors, SSB provides an increase in overall efficiency.
In fact, it can be demonstrated that, compared to an AM transceiving system, an
SSB system using similar components can provide an effective 9-dB increase in system
performance.
Posted September 13, 2019
|