November 1961 Radio-Electronics
[Table of Contents]
Wax nostalgic about and learn from the history of early electronics.
See articles from Radio-Electronics,
published 1930-1988. All copyrights hereby acknowledged.
|
The
International Geographic
Year (IGY), which ended up lasting about 18 months, ran from July 1957 through
December 1958. One of its main purposes was to explore and quantify the nature of
the electrically conductive region of the Earth's atmosphere, within which and through
which future sounding rockets, orbiting satellites, and deep space probes would
operate. Sputnik launched at the end of 1957, and Echo (embarrassingly) launched
in mid-1960. Both professional and amateur radio operators had already ascertained
significant clues about the ionosphere's characteristics by observing its affects
on electromagnetic signals. Direct, in-situ measurements confirmed some beliefs
while disproving others. More than 65 years later, we know much significantly more
about the ionosphere, having sent thousands of craft - including many manned - into
and through it. When this Nature's Invisible Radio Mirror article appeared in a
1961 issue of Radio-Electronics magazine, people were already personally
benefitting from knowledge gained. See also in this issue
New Link in U.S. Defense - Pacific Scatter.
Nature's Invisible Radio Mirror
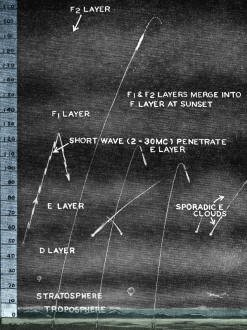
Fig. 1 - The Earth's atmosphere.
Between 40 and 600 miles above the earth is an atmospheric layer
- the ionosphere - that makes much of the world's long-distance radio communications
possible.
By James F. Van Detta, WA2FQZ
A teen-ager slumps into an easy chair and contentedly sips his soft drink as
he loudly taps his feet in time with a strident rock 'n' roll tune from a broadcast
receiver. An amateur radio operator sits before his home-made transmitter and talks
with a fellow ham on the other side of the earth. A young child sits very close
to the television set and gleefully claps his small hands as mild-mannered Huckleberry
Hound jogs nonchalantly across the screen. At a desolate and un publicized outpost,
an alert missile-tracking specialist listens intently to a signal from space as
he carefully tracks an American rocket shot.
All these are instances of electro-magnetic radiation or "radio waves," but only
one, the amateur's contact with the other side of the earth, involves nature's invisible
radio mirror - the ionosphere.
A certain dictionary coyly defines the ionosphere as the ionized layers that
constitute the outer regions of the earth's atmosphere. Such a definition is correct,
but not very informative. Let's look further.
Fig. 1 is a simple representation not drawn to scale, but you can easily see
that the lowest layer of the atmosphere is the troposphere. It extends about 6 miles
above the earth and is known as the "weather layer" because it's within this comparatively
narrow region that the earth's weather exists.
The stratosphere is directly above the troposphere. It extends to a height of
about 40 miles. This is a region in which the air temperature remains practically
constant and doesn't decrease with height as it does in the troposphere where the
average temperature decreases about 3.5° each 1,000 feet.
The ionosphere begins above the stratosphere and goes up to at least 600 miles.
Until recently, it was believed that the ionosphere extended to about 250 miles;
but earth satellites have given us information to indicate that 250 miles is a much
too conservative figure.
"So what causes the ionization in the ionosphere?" you may ask impatiently. The
answer is "The sun's rays." For example, an ultraviolet ray from the sun (the kind
that causes your skin to tan and sunburn) strikes a particle of air high up in the
outer atmosphere and knocks an electron from it. Since an ion is an electrically
charged particle that has fewer or more electrons than normal, the obvious result
of such a collision is a positive ion (a particle minus an electron) and, of course,
a free electron. Countless such collisions produce innumerable ions and free electrons,
and thus an "electrified," or ionized, layer is produced.

Fig. 2 - An example of skip (sky-wave) propagation.
Ionosphere Layers
The D-layer is directly above the stratosphere. It may be anywhere between 30
and 55 miles wide - the height, depth and degree of ionization on any ionosphere
layer depend upon the time of day, the season of the year and the amount of sunspot
activity.
The D-layer forms in a fairly dense region of the atmosphere, so the particles
that are ionized there recombine rapidly. Since the amount of ionization is directly
related to the amount of sunlight, ionization in the D-layer is greatest around
noon and disappears completely after sundown.
The D-layer is not useful for communications. The dense ionization in this layer
absorbs a great deal of the energy of certain radio waves - say those below 10 mc.
The degree of absorption is inversely proportional to the frequency. Therefore,
on waves above 10 mc, for example, absorption is relatively small. Many short-wave
listeners and radio amateurs curse the D-layer as the culprit when the 80-meter
amateur band (3.5-4.0 mc) "goes dead" for a while around noontime.
The E-layer is the lowest one that acts as a radio mirror and is useful for long-distance
communication. Shown in Fig. 1 at a height of about 70 miles, it may be anywhere
between 55 to 90 miles.
Although the air particles in the E-layer are somewhat less numerous than in
the D-layer, the region is still relatively dense; the ions and electrons recombine
rather rapidly, and ionization is minimum at midnight. At maximum ionization, near
noon, the E-layer, like the D-layer, absorbs energy from low-frequency radio waves.
But, unlike the D-layer, the E-layer reflects some radio signals. At high noon,
for example, the 80-meter amateur band (3.5-4.0 mc) previously mentioned may be
useless because these frequencies are completely absorbed in the D-layer; but the
40-meter amateur band (7.0-7.3 mc), despite some absorption in the D-layer, may
provide communication for hundreds of miles due to E-layer reflection.
The F1-layer, at its average height of about 125 miles, exhibits most of the
characteristics of the E-layer.
The highest and most useful layer of the ionosphere is the F2-layer which sits
at an average height of about 200 miles. Because the air in this region is extremely
thin, the ions and electrons in it recombine very slowly. Ionization in this layer,
therefore, is not as directly related to sunlight as in the other layers. A short
while after noon, the layer reaches maximum ionization. Afterward, ionization gradually
decreases to a minimum just before sunrise. The F2-layer acts as a highly effective
radio mirror for long-distance communication.
After sundown, the F1-layer combines with the F2-layer, which then drops down
in height. Keep in mind that Fig. 1 illustrates a daytime condition.
Radio Propagation
Radio propagation using the reflective characteristic of the ionosphere is termed
"ionospheric skip" because the signal skips over large areas as it hops on its way.
Referring to Fig. 2, note that the skip distance is the distance from the transmitter
to the point where the signal again returns to earth. The signal may then be bounced
upward to be reflected once more from the ionosphere and thus complete another skip.
Such multihop propagation makes world-wide radio communication possible. Because
the ionospheric skip signal is reflected from the sky (that is, the ionosphere),
this is also called sky-wave propagation.
Obviously, the ionospheric skip signal jumps over certain places and cannot be
heard there. If you would like dramatic proof of this, listen in on the 10-meter
amateur phone band (28.5-29.7 mc) sometime when it is "open." You'll find that you
can generally hear only one side of the conversation - because the sky wave of the
other station skips right over your location!
Ionospheric skip or sky-wave propagation is but one type of wave propagation,
of course. The rock 'n' roll teenager mentioned at the beginning of this article
probably couldn't care less, but it is primarily ground-wave propagation that provides
him with the programs of standard broadcast stations. Ground-wave (or surface-wave)
propagation refers to waves that cling closely to the earth as they travel. The
earth's surface absorbs much of the wave's energy, limiting this type of propagation
to about 100 miles for standard broadcast transmissions.
The young child mentioned earlier can enjoy his favorite television program because
of line-of-sight propagation, which is just about what the name implies - the waves
travel in a rather direct line from the transmitter to the receiver. This type of
transmission applies to frequencies from about 30 mc on up; ordinarily the ionosphere
does not reflect such waves. If they are directed at the ionosphere, they simply
travel off into outer space.
Since signals at frequencies higher than 30 mc go through the ionosphere and
on into space, the so-called Age of Space Communications is possible. The missile-tracking
expert probably had radio equipment for frequencies from 1,000 to several thousand
megacycles (a megacycle, remember, is 1,000 kc).
While space communications is a very romantic and promising field, ionospheric
skip still provides the means for much of the world's long-distance communication,
as well as providing radio amateurs and short-wave listeners with fascinating hobbies.
The big problem, with ionospheric skip propagation is that it is erratic. For
example, certain eruptions on the sun cause ionospheric "storms" and a disturbance
of propagation conditions. At other times, mysterious radio blackouts may disrupt
communication for a few minutes or many hours.
Since so many variables are involved in ionospheric skip propagation, it is extremely
helpful to know the maximum usable frequency (MUF), the highest frequency that can
be used for communication over a given path. The MUF for any given transmission
path is related to the sunspot cycle in that the MUF tends to be high during sunspot
maximum and low during sunspot minimum. Since we are now in a declining sunspot
cycle that will probably reach a minimum in the 1962-63 period, you may expect,
for the next few years, that the higher short-wave frequencies-say, above 15 mc
- will be less and less useful for long-distance communication. Fortunately for
all who are interested, several very helpful sources of propagation information
are readily available to anyone.
Information Sources
Charts that predict the MUF for any transmission path on earth are issued by
the Central Radio Propagation Laboratory of the National Bureau of Standards. These
charts, called "CRPL-D Basic Radio Propagation Predictions," cover both E- and F2-layer
propagation, are issued monthly and provide predictions for 3 months in advance.
The cost is 15c per copy, or a year's subscription (12 issues) for $1.50. Circular
465, "Instructions for the Use of Basic Radio Propagation Predictions," tells how
to use the charts and costs 30c. Both the charts and circular are available from
Superintendent of Documents, US Government Printing Office, Washington 25, D. C.
Radio-Electronics publishes a monthly Short-Wave DX forecast which predicts the
optimum short-wave broadcast frequencies for the coming month (see page 55). This
forecast is prepared from the CRPL-D charts.
Another Source of helpful propagation information is the CRPL series Jb' reports
issued every Wednesday by the CRPL Radio Warning Service at Boulder, Colo. These
weekly reports, issued in postcard form to facilitate mass distribution, include
a forecast of geomagnetic conditions based on solar and related data and a record
of past geomagnetic activity. Since magnetic disturbance and radio disturbance are
strongly correlated, the Jb' reports provide a valuable basis for assessing future
propagation conditions.
Send requests for the reports to the CRPL Radio Warning Service, US Department
of Commerce, National Bureau of Standards, Boulder, Colo. charge is $4.00 per year
in US, Canada or Mexico. All other countries $5.00.
Still another source of reliable propagation information is station WWV, operated
continuously by the National Bureau of Standards, Washington, D. C. It operates
on frequencies of 2.5, 5, 10, 15, 20 and 25 mc. At 19 1/2 and 49 1/2 minutes after
each hour, propagation information applying to transmission paths over the North
Atlantic is given in International Morse code. This information consists of a letter.
A number, also in code, follows the letter and indicates the expected propagation
conditions during the following 6 or more hours. Forecasts are revised four times
a day, at 12 midnight, 7 am, 12 noon and 6 pm, EST.
The chart shows the letters and the numbers and their significance, as well as
the code for each. The code is sent slowly during these broadcasts. Even if you're
a short-wave listener who doesn't know a thing about the code, you'll quickly find
that you can make out the propagation forecasts. Remember that the forecast consists
of one letter and one number, and this code combination is repeated several times
before the call sign and time are given by voice.
Station WWVH in Hawaii, operating on 5, 10, and 15 mc, has similar forecasts
for the North Pacific at 9 and 39 minutes after the hour.
So whether ham or short-wave listener, get the CRPL charts and the Jb' reports,
check the propagation forecasts on WWV, and then roll up your sleeves and go after
those "rare ones." And the best of dx to you, via nature's invisible but wonderful
radio mirror - the ionosphere!
Posted July 17, 2024
|