April 1958 Radio-Electronics
[Table
of Contents]
Wax nostalgic about and learn from the history of early electronics.
See articles from Radio-Electronics,
published 1930-1988. All copyrights hereby acknowledged.
|
"Ground is ground the world
around," is an oft repeated saying when talking about making electrical connections
to Earth ground. In a general sense that is true, especially when referring to electromagnetic
radio signals and antenna systems that are in some manner dependent on the common
connection. However, when you are working within the confines of a localized electronic
circuit such as on a printed circuit board or inside a chassis, there is no guarantee
that without proper precautions ground is not at the same potential everywhere.
Poor (high impedance) soldered, crimped, and bolted connections are among the prime
offenders that cause voltage differentials to arise between points intended to be
equipotential. RF frequency signals are particularly sensitive to even a minor divergence
from the designed impedance because standing waves and reflected signals can and
will generate signal distortions. This brief article runs through some of the more
common causes of grounding problems. Anyone working with older electronic equipment
might find it especially useful.
Uncommon Ground Difficulties
Grounding isn't always easy. Even an inch of
wire can cause trouble
By A. R. Clawson
Technical workers, experimenters, hobbyists and even some engineers consider
an electronic chassis a common ground. This common-ground idea often proves false.
Case histories, including causes and solutions, of a few uncommon ground troubles
are presented here.
Even the smallest part of a metal chassis presents some opposition to the flow
of current. This is its impedance and for DC is equal to the ohmic resistance. There
is no need to get involved in the calculation of impedance, but we must look into
some of its effects.
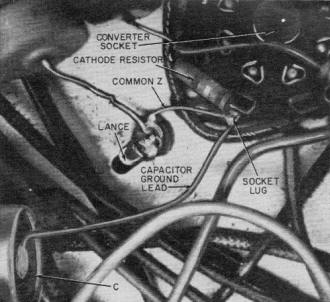
Fig. 1 - Hum modulation, due to an inch of wire.

Fig. 2 - Equivalent circuit showing filter capacitor return
to lug and then along common impedance of converter stage.

Fig. 3 - Common and nearby grounding of horizontal oscillator
bypass and IF bypass capacitors results in jitter.

Fig. 4 - Mutual interaction of ground currents from nearby
ground points.

Fig. 5 - Capacitive coupling between a hot chassis and a
hot conductor; a - actual circuit; b - equivalent circuit.

Fig. 6 - a) In-line layout minimizes chassis current interaction;
b) bent layout, with possible regenerative or degenerative feedback.

Fig. 7 - Poorly soldered lug introduces a high common impedance
between the common joint of two capacitors returning to ground.

Fig. 8 - The joint in Figure 7 after resoldering.
To AC the impedance is a combination of AC resistance and net reactance - primarily
inductive reactance. AC resistance is not the same as DC resistance, but always
greater. The skin and other effects add to the DC resistance. This may increase
the resistance factor by as much as 12 times at television frequencies.
The other component of impedance, the reactance, may reach rather high values
with increasing frequency for inductive circuits. A 1-inch piece of ordinary hookup
wire can have 4 ohms of inductive reactance at 30 mc! Yet its ohmic (ohmmeter) resistance
is too small to measure with an ordinary meter.
Hum Modulation
An example of this type of trouble turned up in an ordinary broadcast receiver.
The set had a bad case of hum modulation, indicated by no hum when the set was tuned
off station.
Troubleshooting was rapid since the hum increased as the higher-frequency stations
were tuned. The variation ruled out the if stages, leaving only the converter. The
trouble was found to be an incorrectly grounded lead from an electrolytic capacitor.
Fig. 1 shows a wire running from a ground lance (punched-up lug) to a socket
lug of the converter stage. Note the cathode resistor of the tube and the ground
return of the electrolytic capacitor returning to the socket lug. Both cathode current
and capacitor current flows through the wire from socket lug to lance.
Capacitor current has two components of interest: reactance flow equals the ripple
voltage divided by the capacitor's reactance; AC leakage current, equal to ripple
voltage divided by the electrolytics leakage resistance. There is also a DC component
of no concern here.
The wire offers some impedance at 120 cycles - we need not worry about calculating
it. But we do have to note that there is a voltage drop:
E = I x Z (or E = IZ)
where I is the combined alternating current and Z the small but definitely present
impedance at 120 cycles.
Fig. 2 shows the circuit. The wire and, to a lesser extent, the lance form
the common impedance, with some small contribution from the socket lug, making Zcommon.
Its equivalent circuit is shown as an insert. The leakage resistance and capacitive
reactance are shown as resistances. The voltage developed, Ecommon is
applied to the cathode of the tube in series with a cathode resistor. The ripple
voltage modulated the cathode of the converter in this instance.
The value of Zcommon increased with increasing frequency, permitting
more of the developed hum voltage to be effective. This follows from the formula:
XL = 2πfL
Inductance L remained constant while frequency f increased 3 times from 500 to
1,500 kc, with a similar increases in X1 - the reactance-and the impedance of Zcommon
at these frequencies.
Moving the ground-return wire of the electrolytic to the lance, where it had
been prior to electrolytic replacement in another shop, cured the hum. Just an inch
of common ground wire caused all the trouble!
False Sync Pulses
Feedback of sweep or sync into the video or pix if or front end can result in
an unwanted and false sync pulse. Sweep oscillators, even though afc-protected,
may try to lock on the added pulse with resultant symptoms of jump, jitter, and
sometimes even vertical roll.
Typical of this class (see Fig. 3) is the case of horizontal oscillator
feedback, or injection. A tubular ceramic (C1), a horizontal oscillator bypass carrying
sweep currents to ground and disc capacitor (C2) were grounded to the same ground
lance (lug). The lug acted as the common impedance. Feedback of horizontal oscillator
pulses into the picture if resulted in jitter whenever the horizontal frequency
drifted ever so slightly. The design error was corrected in later production runs.
Not all feedback was due to the common impedance of C1 and C2's common ground
lance. Some feedback was caused by the current of C1 intermingling with the chassis
current of C3, a disc if bypass capacitor. The common impedance was the sheet-metal
chassis proper! The solution was to move C3's ground to the same point as C1.
Fig. 4 shows what happens. Tube V1 has a bypass C1 and tube V2 has as its
bypass C2. The tubes may have different functions like the sweep oscillator and
if just mentioned. The bypass capacitors normally return their current through the
chassis to the cathode of the tube. Heaviest current flow is between the ground
point of the capacitor and the cathode or its bypass capacitor. Not all the current
goes in a straight line however, but forms a sweeping motion in accordance with
the low but existing chassis impedance. The lines in Fig. 4 enclose approximately
equal areas of current flow and equal chassis impedance.
Note that the lines of flow (flux) of capacitor C1 intermingle with those of
C2. The result is a voltage, similar to that developed in the hum modulation case.
This time it is in the chassis instead of a wire. The voltage is small and can be
disregarded in many instances. Sometimes, this voltage may inject another voltage,
a false sync pulse, for example. Degenerative or regenerative feedback may also
occur.
Worthy of note is the control of chassis currents and common impedances by chassis
openings. If a row of slots or holes is punched between the sockets of V1 and V2
(Fig. 4), the common impedance is interrupted to a large extent. Where rerouting
the capacitor is not feasible, this might be a satisfactory solution.
Capacitive Reactance
Cases occur of capacitive reactance between a wire or other conductor and a hot
chassis. The chassis may be carrying a large current at a high frequency and, if
a conductor is too close, a very low feedback path may exist due to the capacitance
formed. The reactance will be low at high frequencies according to the capacitive
reactance formula:

Fig. 5 is a sketch of such a wire close to a chassis. Feedback of deflection
yoke currents into tuner (front-end) shields has occurred by this method. The remedy
is redressing the yoke leads. Commercial equipment does not lend itself to layout
changes but such information is helpful to those that make their own. Careful layout
can avoid trouble.
As an example, Fig. 6-a shows a straight-line amplifier - the tube sockets
in line. Given the same components, the in-line amplifier can yield greater gain
than the bent amplifier of Fig. 6-b. The reason is that the bending crowds
the current flux (flow) into the corner, and input and output currents of the tube
at the bend mix in the common chassis impedance. The result may be instability.
Tube sockets are mounted in holes in the chassis. A ground point may be selected
on the opposite side of the tube. For example, in Fig. 4, the common impedance
between C1 and C2 can be greatly lessened by using new ground points at X. The socket
holes interfere with current flow toward the other tube for each capacitor. This
is more effective than the slot method.
Another way to keep ground currents in line is to increase the conductivity of
the chassis or ground in the desired direction, thereby diverting current from undesired
paths. A strip of braid may do the job very nicely. In Fig. 1. paralleling
the inch of wire with braid would probably reduce the common impedance to where
the hum modulation would not be objectionable. However, moving the capacitor lead
was the easy way.
A sheet of metal, riveted, soldered, or bolted to the chassis, can serve to divert
currents by lowering the impedance.
Fig. 7 shows the circuit formed by a poorly soldered connection between
a ground lug and two capacitors making; a return at that point. Fig. 8 shows
the actual parts after resoldering.
The impedance Zcommon should have been nearly zero ohms in this case,
but actually was about 0.5 megohm. The large electrolytic and an agc filter capacitor
in Fig. 8 were grounded at the lance. Due to the common impedance, the power
supply ripple fed into the age line practically without hindrance-the only opposition
was the reactances of the capacitors themselves.
Heater Grounds
Common practice uses the chassis as one return leg for the heaters of all tubes.
These can intermingle with signal currents to produce unwanted effects. Heaters
should be connected directly to their own ground rather than to another socket lug
because the common impedance may become a trouble spot that will be difficult to
localize. Assume the wire in Fig. 1 is the common return of a heater and a
cathode - the effect might resemble cathode-to-heater leak-age and no amount of
tube substitution will remedy such a situation.
Happy grounding!
Posted May 17, 2023 (updated from original post
on 5/25/2014)
|