Aug/Sep 1940 National Radio News
[Table
of Contents] These articles are scanned and OCRed from old editions of the
National Radio News magazine. Here is a list of the
National Radio News articles I have already posted. All copyrights are hereby acknowledged. |
1940 was a big year in
the commercial broadcast industry because it was when the FCC began licensing stations
for FM operation. Amazingly, that was only four years after
Edwin Armstrong first
came up with his frequency modulation scheme - fast moving for the government. Simultaneously,
equipment manufacturers were cranking out transmitters, receivers, antennas
(new frequencies), writing installation and operation
guidelines, training servicemen, and doing scores of other vitally important tasks.
The advent of FM was considered a very significant technical improvement because
of immunity to electrical noise interference. If for no other reason, you should
look at this National Radio News magazine article to see the photo of the megavolt artificial lightning discharge
created to test and demonstrate FM's tolerance of such phenomena.
FM Receivers and Their Alignment

Joseph Kaufman
By Joseph Kaufman
N. R. I. Director of Education
With about thirty frequency-modulated (f-m) broadcasters in operation and one
hundred station permits granted, and with the F.C.C. formally acknowledging that
f-m signals will serve public interests, localized radio broadcasting enters a new
spectrum, namely 42 to 50 megacycles.
Even the most enthusiastic admit that with forty-five million amplitude-modulated
(a-m) receivers in use today in these United States, wide public acceptance of f-m
will take from five to ten years. At this early date, well-informed receiver merchandisers
say that the evils which f-m is said to overcome are not as "wicked" as most people
would like to have us believe; hence they argue that f-m may not be the "whirlwind"
that initial publicity leads us to believe.
However, it cannot be denied that f-m provides an almost noise-free signal, to
a degree not attainable with the a-m system. To be sure, local man-made interference
has been greatly reduced in our present system, but atmospheric and lightning disturbances
still affect a-m receivers. With f-m transmission, however, reception can be noise-free
even during local electric storms. Its victory over noise is the greatest appeal,
but f-m can provide high fidelity with depths of volume never before considered
feasible for a-m.
Whether the public as a whole wants true fidelity and natural reproduction is
still a highly debatable subject; a great deal of evidence indicates that after
years of ear subjugation to false reproduction, broadcasting has developed an ear
for reproduction peculiar to radio.
How f-m will be accepted by the public, only time will reveal; it is here, however,
and offers a real opportunity to trained radio technicians.
Review of Fundamentals
Before we go on to consider the f-m receiver, a review of the differences between
amplitude and frequency modulation deserves consideration.
With amplitude modulation, a basic r.f. signal called the carrier is increased
and decreased in amplitude, in accordance with the sound intelligence that is to
be conveyed. The basic r.f. signal amplitude is never increased more than twice
the carrier level, and never reduced to such an extent that an r.f. signal does
not exist for an instant. Amplitude modulation produces side frequencies with the
highest audio frequency determining the band width. For example, if a 10-kc. audio
signal is the limit, modulated on a 1,000-kc. carrier, the side frequencies will
extend from 990 kc. to 1,010 kc.
 General Electric frequency modulation receiver
undergoing comparative listening tests while subjected to million volt lightning
discharge.
With frequency modulation, the amplitude of the r.f. carrier remains fixed at
all points in a given communication system. When no sound is being transmitted,
the frequency of the signal is a definite value which is often referred to as the
"resting" frequency. This frequency is increased and decreased in accordance with
the level (or volume) of the sound being transmitted.
Let us look at it this way; sound is the result of condensation and rarefaction
of air particles. Condensation results in a dense group of air particles, and rarefaction
results in a below-normal amount of air particles. We could arrange to increase
the radio frequency for conditions of condensation, and decrease the frequency for
rarefaction.
There must be a limit to the frequency swing from the resting value, depending
on the maximum sound level intended, and this range is referred to as the frequency
deviation. Thus, for the loudest sound to be transmitted, the swing could be limited
to 75 kc. Since the frequency is varied above and below the resting frequency by
this value, the total deviation will then be 150 kc. For example, if the resting
frequency is 43,000 kc., for the loudest sound the frequency will swing from 42,925
kc. to 43,075 kc. Should this loudest sound have a 1,000-cycle pitch, the r.f. signal
will vary from 43,000 to 42,925 to 43,075 and back to 43,000 kc., one thousand times
in a second. If this 1,000-cycle sound has a lower level, the swing could be from
42,995 to 43,005, one thousand times a second.
In frequency modulation, the instantaneous frequency corresponds to the sound
level at that instant, and the rate at which the frequency is varying above and
below the resting value is the pitch of the sound.
As far as fidelity of transmission is involved, the deviation can be any value;
in fact, equally as good fidelity can be obtained with an overall deviation of
20 kc. as with 150 kc.
For maximum elimination of noise. however, a large frequency deviation is desirable.
A noise pulse received along with the f-m signal affects the instantaneous amplitude
by creating peaks on the r.f. signal, and also affects the instantaneous frequency
of the signal.
As we will see later. the amplitude peaks of noise are removed by the "limiter"
in the f-m radio receiver, but any instantaneous change in the signal frequency
will introduce volume pulses after the f-m signal is converted to amplitude changes.
If a large frequency change is employed to produce an appreciable change in sound
level, the frequency change due to a noise will normally have little effect in producing
noise interference. On the other hand, if full range in volume is produced with
a small frequency deviation, the frequency change produced by noise pulses will
be quite apparent.
Essential Stages in an F-M Receiver
Once you understand the basic principles involving f-m receivers, you will find
these new sets are no more difficult than ordinary sets.
As you will shortly see, no radically new circuits are used in a f-m receiver.
Conventional vacuum tube circuits, designed to meet special requirements are predominant.
The superheterodyne circuit is employed, usually with a stage of r.f. ahead of the
frequency converter, and with one or more i.f. stages following the converter.
After sufficient amplification has been obtained, a stage which will convert
f-m to a-m is required. This modulation converter must be followed by a normal amplitude
type of detector.
There is, however, a modulation converter which also detects at the same time.
The discriminator circuit used in automatic frequency-controlled receivers will
produce positive and negative voltages, the instantaneous voltage depending on the
deviation in frequency from the reference frequency. Thus, f-m can be converted
directly to audio signals by an a.f.c. discriminator circuit.
Between the frequency discriminator and the last i.f. stage, a special tube circuit
(called the limiter) is introduced. Although its elimination would not prevent f-m
reception, its use definitely results in the unique features which make f-m transmission
superior to a-m. A limiter removes all amplitude noise pulses, so the discriminator
output contains negligible noise signal. The limiter keeps all signal amplitude
levels below the permissible swing of the discriminator, thus preventing amplitude
distortion. The discriminator also operates so as to favor the desired (stronger)
signal and suppress the weaker undesired signal. Finally, the limiter supplies a
negative d.c. voltage proportional to carrier intensity, hence it is used as an
a.v.c. source.
Following the discriminator is a potentiometer which serves as a volume control;
its output feeds into a conventional a.f, amplifier and loudspeaker. For high fidelity,
both the a.f. amplifier and loudspeaker must be designed to have essentially uniform
response over a wide range of audio frequencies. The loudspeaker system usually
consists of a low-frequency unit and a high-frequency reproducer, acoustically compensated
for high-fidelity reproduction.
For some time, f-m receivers will also include circuits for a-m reception. The
preselector and oscillator coils for any a-m band will be switched into the circuit;
the same switch will also switch in the 42-50 megacycle coils, and in all probability
will also introduce the low-capacity variable condensers required for band-spread
tuning. Such a combination f-m and a-m circuit is shown in Fig. 1.
In each i.f. section of the circuit in Fig. 1, the secondary of the i.f.
transformer for a-m (usually about 456 kc.) will be connected in series with the
secondary of the f-m i.f. transformer (usually about 1 to 7 megacycles). By connecting
the secondaries in series and by preventing any mutual coupling, one i.f. transformer
will have negligible impedance at the other frequency, hence its presence will not
interfere with amplification of the desired frequency.
The primaries of the a-m and f-m i.f. transformers will be switched into the
plate circuit of the tube to which they are connected, as shown in Fig. 2.
If this is not done, transfer through both transformers would exist, and the selectivity
of the circuits would be lost.
The i.f. channel for f-m will employ a number of stages, only one of which is
shown in Fig. 2. This i.f. channel will feed into a limiter-discriminator type
of detector for f-m. The input of the a.f. amplifier will be switched from the f-m
detector to the a-m detector according to the type of reception desired.
F-M Antenna System
The all-wave antenna used for a-m signals is not designed
for high-frequency radio waves. An additional antenna will be desirable. Half-wave
antennas are being widely used; these are connected directly to the input terminals
of the receiver, and these in turn connect to the primary of the 42-50 mc. antenna
coil.
Two antennas will thus be normally used for f-m and a-m reception, as shown in
Fig. 1.

Fig. 1 - Preselector and frequency
converter stages of an a-m and f-m combination receiver.
Any of the antennas found acceptable for television reception can be used. Reflection
will not be a problem. The horizontal antenna should preferably be faced broadside
to the f-m transmitter and adjusted for the least phase cancellation, so the strongest
signal possible is accepted. A horizontal di-pole about 11 1/2 feet long will be
required.
Preselector-Frequency Converter. A stage of amplification preceding the mixer-first
detector is to be expected in an f-m receiver in order to over-ride converter noise.
The tuning condenser will have a low capacity so the L-C ratio of the tuning circuit
will provide high gain. A remote cut-off pentode tube will be used and will be a.v.c.-controlled.
Sufficient selectivity must be embodied in the preselector to eliminate image interference
and i.f. signal interference.
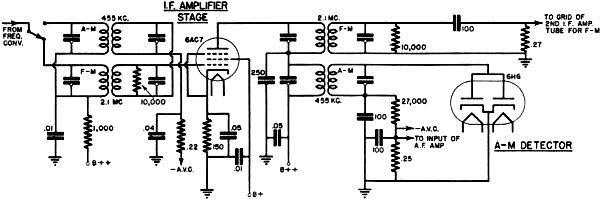
Fig. 2 - Type 6AC7 tube serving
as i.f. amplifier tube for both a-m and f-m reception; primary switching is employed.
Since the output is through independent a-m and f-m channels, switching is required
only at the input of the combined i.f. amplifier
and at the input of the a.f. amplifier.
A conventional pentagrid converter may be employed in the converter stage. although
a triode-pentode tube combination will serve equally well. The local oscillator should
be stable, so that the i.f. amplifier and the discriminator characteristics need
not be made too broad.
F-M Intermediate Amplifiers
These will be of the conventional double-tuned type,
carefully designed for optimum coupling so that a resonance curve with a flat top
and steep sides will result. As we will see later, perfectly flat tops are not required
for strong signals, as the limiter will give the entire r.f. system a flat-top resonance
characteristic. However, sufficient broad response will be required so that amplitude
distortion does not arise for weak signals. To get sufficient broadness in tuning,
either the primary or the secondary of the i.f. transformer will be loaded with
a resistance, about 10,000 ohms. Because of loading and the use of a high i.f. value,
about 1 to 7 mc., low gain will result and at least two i.f, tubes will normally
be required.
Limiter. This stage, important as its functions are, is a simple tube circuit.
An ordinary pentode tube, operating at low plate and screen grid voltages (about
60 volts) and with no initial grid bias, is used. Grid current flows upon application
of an r.f. signal, and this rectified current is made to flow through grid return
resistors R1 and R2 as shown in Fig. 3.
Using low plate voltage causes the plate current to cut off at low negative grid
voltage values. The upper limit of plate current is also kept low by the low plate
current, for the space charge readily prevents the flow of electrons to the plate
when low accelerating potentials exist; excessive. grid current contributes to low
plate current. As a rule, this circuit is designed so that the limiter output does
not vary more than 1 volt for all input r.f. voltages above the saturation value.

Fig. 3 - Typical limiter
and frequency discriminator circuit.
While the limiter circuit prevents excessive rises in the amplitude of the current
output, the grid current in the limiter stage causes the operating point to move
more negative from the no-signal point shown as a in Fig. 4. The operating
point may assume a position such as b, c or d as the input level of the signal increases.
The normal operating point will be somewhere between c and d, a condition for high
signal input. In such a case, the r.f. signal will undergo half-wave rectification,
and plate current will flow for half a cycle or less. Since the plate load of the
limiter (Fig. 3) will be a resonant circuit, the voltage developed across the
tank circuit will have both alternations of the cycle with the tuning circuit possessing
the ability to sustain oscillation at its resonant frequency by virtue of the energy
stored in this circuit.
If noise pulses make the input r.f. signal swing positive beyond point x in Fig.
4, the pulses will be removed by the saturation effect of this limiter; negative
noise peaks will be removed by the cut-off characteristic of the limiter. Of course,
this only occurs when a strong signal is received, and the a.f. system is hence
designed to load the limiter fully for the weakest signal to be received.
If the desired signal fully loads the limiter, causing the operating point to
be more negative than point c (see Fig. 4), a weak signal entering the limiter
will either cause no plate current variation or will reduce the plate current variation
to such a low amplitude that the limiter output resonant circuit will not receive
enough energy to sustain this oscillation. It is possible to design the limiter
so that a desired signal which has twice the amplitude of an undesired signal, both
operating at the same resting frequency, will so completely over-ride the undesired
signal that the latter will not be heard.
The flow of grid current through grid resistors R1 and R2
in Fig. 3 produces a voltage across the resistors which self-biases the limiter
to cut-off or beyond for normal and above-normal signal levels. This negative voltage
may, in some receivers, be used to operate an electronic tuning eye,* or to feed
an a.v.c. voltage to any of the i.f. or r.f. stages which it may be desirable to
control. The resulting a.v.c. action will prevent the limiter from being overloaded
too much on very strong signals.
In passing through the r.f. amplifier of the f-m receiver, the signal is varying
in frequency above and below the resting frequency. If the r.f. system is sharp,
as shown by curve 1 in Fig. 5, the signal amplitude will vary from a to b to
c to d to e and back to a for one audio cycle. To prevent such extreme variation
in amplitude, the frequency deviation would have to be limited so the swing would
be from y to z, or the r.f. system would have to be made much broader by loading.

Fig. 4. Limiter characteristic curve.

Fig. 5. How the limiter flattens the r.f. response.

Fig. 6. The S curve of a frequency converter.
As was previously, pointed out, the limiter in itself causes the over-all response
of the r.f. system to act broad. If the level of the signal is so proportioned that
all signal amplitudes above b (amplitude greater than x in Fig. 4) are in the
saturation region of the limiter, then all such peaks will be removed. The r.f.
amplifier and the limiter together will then have the resonant response portrayed
by curve 2 in Fig. 5. The f-m signal may thus embody wide deviation in modulation.
The r.f. system is never made too sharp for signals which do not drive the limiter
to saturation. Weak signals do not benefit by this action; to receive them with
good response, the r.f. and i.f. amplifiers must be reasonably broad.
Frequency Discriminator.
This circuit does not differ from the circuit used in
automatic frequency control. except that it is designed for the i.f. value used
in f-m receivers. Such a circuit is shown at the right in Fig. 3.
Note that two diode rectifiers are used, each diode being fed with one-half the
voltage of the final resonant circuit. Being a split secondary connection, one diode
input r.f. voltage is 180° out of phase with the other diode input voltage.
At the same time, both diodes get the full r.f. voltage which is present at the
plate of the limiter. When the frequency is off the resting value, as it is during
transmission of intelligence, the phase relationship between the r.f. voltages acting
on each diode varies. As a result one diode gets more r.f. voltage than the other,
and the rectified d.c. voltages differ. The difference in d.c. voltage is the f-m
demodulated signal; its amplitude is proportional to the amount the signal frequency
differs from the resting value and its polarity depends on whether the signal is
above or below the resting value.
Thus, while the f-m signal is varying in frequency due to modulation, the net
d.c, voltage across R3 and R4 in Fig. 3 is changing in
amplitude, with point m becoming alternately positive and negative with respect
to ground. Condenser across R3 and R4 remove all r.f. components.
The discriminator must be designed so that increases and decreases in frequency
from the resting value produce proportional changes in d.c. output voltage, as shown
in Fig. 6. This linearity must extend for the full deviation in frequency.
It is important to standardize on the maximum deviation that will be used, and design
the discriminator accordingly. In fact, the discriminator should be able to handle
even a greater deviation, to take care of the normal drift in the frequency of the
receiver oscillator.
By designing the discriminator for wide frequency deviation, this stage will
function for f-m signals with low frequency deviation. A discriminator designed
for a narrow frequency deviation would distort when an f-m signal with a wide deviation
was received.
Alignment of F-M Receivers
Alignment of an f-m receiver will differ somewhat from the procedures used for
a-m receivers. It may surprise you to learn, however, that this alignment can be
done with standard servicing equipment having suitable ranges.
First, the discriminator will be lined up, A high-resistance d.c. voltmeter,
preferably a vacuum tube voltmeter, is connected across one diode load resistor.
To introduce a signal, connect the service signal generator to the grid-chassis
of the limiter tube. The signal generator should be set exactly to the i.f. value
for f-m, and its output should be as high as possible, about 1 volt. Adjust the
primary of the discriminator transformer for maximum output. Now connect the d.c,
voltmeter across both diode loads, and adjust the secondary of the discriminator
transformer for zero output voltage.
To align the resonant circuit ahead of the limiter stage, connect the signal
generator (still set at the i.f. value for f-m ) to the grid-chassis of the stage
ahead of the limiter. A 0 to 100 micro-ampere meter can be connected in the grid
return of the limiter, or a high-resistance voltmeter (or v.t.v.m.) can be connected
across the grid return resistor which produces the a.v.c. voltage. Adjust the resonant
circuit ahead of the limiter for maximum deflection.
When a peak reading is obtained, the output reading should be high enough to
indicate that the limiter is being saturated. To do this, the signal generator output
should be set up to a high output value. This can be checked by noting the output
across one diode load in the discriminator; increased input to the limiter should
show little rise in output voltage. This condition is essential, for it is necessary
to have the same loading of the limiter on the resonant circuit as would exist in
normal operation. This loading affects the response of the resonant circuit. If
you align this circuit with little load, a different peak setting will result.
Advancing the signal generator one stage at a time aligns each resonant circuit
for maximum limiter grid current or self-rectified d.c. voltage. The i.f. channel
for f-m will be aligned when the signal generator is connected to the input of the
mixer-first detector,
Next is the alignment of the preselector and oscillator. For this adjustment,
the signal generator is connected to the two antenna posts. The oscillator is always
aligned first, and the preselector is adjusted for maximum grid current or voltage
in the limiter. Alignment will, of course, depend upon the type of tracking employed.
One method worth mentioning involves the iron-core coil in the oscillator. The signal
generator and the receiver dial are set at a low frequency (about 42 to 43 mc.),
and the oscillator core aligner is adjusted for maximum output. Then the signal
generator and receiver are set to a high frequency (about 49 to 50 mc.) and the
trimmer shunting the oscillator variable condenser is adjusted for maximum output.
* An f-m receiver should be tuned for least noise, not
for maximum sound level. An electric eye working on peak limiter grid current offers
an excellent tuning indicator.
|