That ultrasonic communications
has not proved to be a reasonable means of transmitting information from one location
to another - even over fairly short distances - is borne out by the obvious lack
of such systems today. With all the technology available in the form of electronics,
mechanics, and software, if it were possible to efficiently and effectively implement
systems of ultrasonic communications, such devices would be as common as the current
plethora of wireless systems. Some early research efforts at ultrasonic communications
were published in a 1945 edition of Radio News magazine. Regardless of
the era, the electromagnetic frequency bands are always deemed to be too crowded
so researchers constantly look for other transmission media. There is one revolutionary
new potential form of remote communications on the horizon:
quantum
entanglement. Still largely an enigma, entanglement communications exploits
an observed property of some subatomic particles to be inextricably linked to each
other with no discernable medium or known mechanism. Albert Einstein referred to
it as "spooky action at a distance." Don't look for quantum entanglement Internet
routers anytime soon, but once the technology comes to fruition, not only will it
mitigate the need for distribution coaxial and optical cable, but also local routers;
ultimately, only a QE modem will be required in each device connected to the ubiquitous
Internet
of Things (IoT).
An analysis of the principal factors and equipment involved in conveying intelligence
at ultrasonic frequencies through mediums such as liquids, gases, or solids.

Fig. 1 - Two types of reproducers (loudspeakers) that can
be used to communicate via a 20 kc. ultrasonic sound beam. (A) The magnetostriction
reproducer and (B) the piezoelectric reproducer. Photographs of these units are
shown in Figs. 2 and 4 respectively.
By Robert G. Rowe
In order to convey intelligence or to "communicate" through a medium, be it a
liquid, gas, or solid, it is expedient first to establish in the medium, as a "carrier",
some form of periodic wave motion which can be propagated to and detected at a remote
point in the medium. Secondly, it is necessary to modify the existence or character
of this wave motion in some pre-arranged, decipherable manner, or "modulate" the
wave motion. Obviously, modifying the existence of the wave may be accomplished
by alternately starting and stopping its generation; whereas, modifying its character
may be accomplished by changing its amplitude, frequency, phase, or velocity. However,
since the velocity of a wave in a homogeneous medium is fixed, the other aforementioned
forms of modulation are relied upon.
In the art of radio communication, such "carrier" waves are electromagnetic in
character, transmitted through the ether at a uniform velocity and modulated to
carry intelligence by code or telephone.
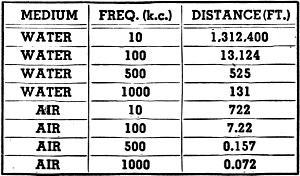
Table 1 - The distance sound travels before its intensity is
reduced to one-half.
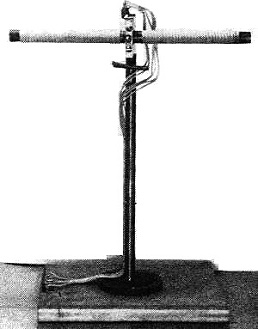
Fig. 2 - The magnetostriction reproducer.
In the art of sonic communication, such carrier waves are "compressional" in
character, wherein the propagating medium suffers a sort of rectilinear deformation,
which may be transmitted through various liquids, gases, and solids at velocities
determined by the characteristics of the medium, and modulated to carry intelligence.
While electromagnetic and sound waves may be essentially different in character,
they are alike in that they may each be propagated in suitable media. Quite obviously,
the most universal form of sonic communication may be exemplified by a conversation
between two or more people. The vocal cords, as well as the configurations and appurtenances
of the oral cavity, cooperate to represent the compressional wave generator, which
is both amplitude and frequency modulated. The air represents the gaseous medium
through which such modified compressional waves are transmitted at a velocity of
some 1100 feet-per-second. The human ear represents the receiver, which is capable
of detecting and, perhaps, demodulating these compressional waves.
In the art of ultrasonic communication, so designated by virtue of the fact that
the human ear will not respond to the higher frequencies, such waves still are compressional
in character and may be transmitted through liquids, gases, and solids. It will
be appreciated that ultrasonic communication, while not popularized, has been known
and used for many years. The early work of Langevin, Florisson, and others describes
and discloses means and apparatus to communicate through a water medium via ultrasonic
waves. Part of this work was directed toward submarine detection and signaling,
as well as echo depth sounding, in which the principles involving the use of compressional
water waves are truly the forerunners of the present-day principles involving the
use of electromagnetic "ether" waves for radar. Early forms of "absolute altimeters"
for avigation also employed sound waves, but their velocity was too low to render
them practical for the vehicular velocities encountered in practice.
Compressional waves, in passing through any medium, are absorbed to an extent
dependent upon the frequency of the waves, the nature of the medium, and the distance
travelled. All mediums have a certain "compressibility" and "viscosity" whereby
all of the compressional energy imparted to displace the medium is not returned
to the wave but is partially transformed into heat. From Rayleigh1, the
amplitude A of plane waves at a distance x from the generator, is:
Ax = A0e-KN²X
(1)
where Ao is the original amplitude, e is the base of the natural logarithms
(2.718), K is a coefficient depending upon the density, compressibility, and viscosity
of the propagating medium, and N is the frequency of the wave motion. By inspection,
the amplitude of the wave at a distance is inversely proportional to the square
of the frequency, so that high frequency compressional waves fade out in much shorter
distances than low frequency waves.

Fig. 3 - (A) Mechanical arrangement of the mechanically
resonant microphone. (B) Cross section view of the dynamic reproducer. Photographs
shown in Figs. 6 and 5 respectively.
The factor K for a water medium has such a relatively low value that appreciable
distances may be covered by compressional water waves at frequencies of from 20
to 50 kilocycles. The factor K for an air medium has a much higher value so that
air distances must be measured in feet rather than in miles. The relative efficiency
of the two mediums, as well as the effect of frequency, may be seen by examination
of Table 1, which shows the approximate range at which the original sound intensity
has been reduced to one half2.
A redeeming feature of ultrasonic sound waves, like high frequency electromagnetic
waves, is that they are easily directed and formed into a cone or beam by conveniently
small radiators. Provided that the vibrating surface producing the wave is moving
like a piston with all surface elements in phase, the directivity of the reproducer
may be roughly calculated from
(2)
where θ is the half-apex angle of the cone, λ is the wavelength
and r is the piston radius3.

Fig. 4 - The piezoelectric reproducer.

Fig. 5 - Dynamic reproducer.
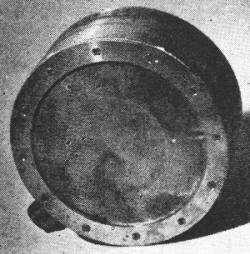
Fig. 6 - Mechanically resonant microphone.

Fig. 7 - When sound-waves along paths D1 and
D2 arrive at the receiver out of phase, as can occur as shown in diagram,
fading and phase distortion occur.
With the foregoing considerations in mind, various apparatus for communicating
via a 20 kilocycle ultrasonic sound beam has been constructed and employed. The
frequency generator to produce the carrier wave consists of a commercial audio oscillator
and ten-watt audio amplifier. In order to amplitude modulate the carrier wave, provision
is made for plate modulating the output tubes of the ten-watt amplifier, in accordance
with conventional AM radio transmitter practice.
Three general types of reproducers or "loudspeakers," namely magnetostrictive,
piezoelectric, and dynamic, have been used. With regard to these reproducers, magnetostriction
refers to that phenomenon in which there is a change of length in a bar of ferromagnetic
material attending magnetization. If the magnetic field is alternating (at 20 kilocycles
in this case), the amplitude of longitudinal vibration in the bar will be maximum
when the frequency of the applied field is equal to the fundamental elastic period
of the bar. In accordance with the formula:
(3)
where f is the frequency in cycles per second, l is the length of the bar in
centimeters, E equals it's modulus of elasticity in dynes per-square-centimeter
and d its density in grams per-cubic-centimeter, the length of a bar for resonance
at 20 kilocycles is approximately 5 inches. This reproducer is shown in Figs. 1A
and 2, clamped for support at its nodal midpoint and strongly magnetized by an additional
direct current magnetic field. In general this simple type of magnetostriction reproducer
is unsatisfactory because of extreme eddy-current heating. Further, this particular
bar, having an o.d. of one-half an inch, has a poor match with the air load. Better
loading may be obtained with the addition of a larger diameter thick disc at one
end.
The piezoelectric reproducer, illustrated in Figs. 1B and 4, consists in a 3.5
megacycle X-cut quartz plate cemented between two identically dimensioned sections
of steel rod. In order to determine the resonant frequency, the required overall
length of this steel-quartz-steel "sandwich" may be approximated from the previously
mentioned formula, because the velocity of sound in quartz and steel is about the
same. This unit has an inherently high impedance requiring a high driving voltage
and necessitating the incorporation of a special matching transformer as used with
piezoelectric instantaneous record cutting heads. The high voltage appearing across
the quartz engenders difficulties in mounting the reproducer.
The dynamic reproducer, illustrated in Figs. 3B and 5, consists essentially of
a small permanent magnet loudspeaker with the cone replaced by a resonant brass
disc one-quarter inch thick and two inches in diameter, pinned at its center to
the central pole piece of the permanent magnet, and carrying the voice coil. This
unit has given excellent service in practice with relatively high output.
Various receivers for receiving the 20 kilocycle sound waves have been employed,
all of them requiring some type of microphone, a carrier amplifier, a demodulator,
a carrier filter, and an audio system, as in conventional radio receivers. Two different
microphones have been successfully used, both being the Rochelle salt crystal type.
The first unit is an unmodified commercial crystal microphone (Astatic WR-20) having
dual crystals and diaphragms, having good response in the 20 kilocycle region. The
second unit, shown in Figs. 3A and 6, employs a stretched foil diaphragm with a
small Rochelle salt crystal cemented directly to the inside face. Provision is made,
as illustrated, for varying the tension on the diaphragm to permit adjustment for
some mode of mechanical resonance at 20 kilocycles. This mechanically "tunable"
microphone has considerably higher output on equivalent sound intensities than the
standard commercial unit, with the added feature of tending to reject lower, non-resonant
frequencies.
Two types of receivers, one rather unusual in design, have been employed. The
first type consists of several stages of tuned audio amplification, followed by
a diode detector, carrier filter, and audio amplifier. As an interesting experiment,
in an effort to improve sub-harmonic rejection, improve selectivity, and eliminate
the necessity for a 20 kilocycle carrier filter, another receiver was designed in
which the output from the microphone is successively doubled to 160 kilocycles,
amplified with a two-stage 160 kilocycle r.f. amplifier, demodulated, and passed
to the conventional audio system. As expected, in this particular arrangement, rather
severe audio distortion is caused by the carrier doublers if a high percentage of
modulation is used. In subsequent experiments it is proposed to use either push-push
doublers or full-wave rectification to elevate the carrier to the higher frequency.
With the frequency raised to around 160 kilocycles, standard 175 kilocycle i.f.
transformers provide conveniently packaged, high-Q tuned circuits for amplification.
Up to the present time, out-of-door tests with the disclosed equipment have not
been made, but experiments which have been performed are of great interest. The
20 kilocycle carrier has been modulated with both tone and phonograph signals and
received exceedingly well over distances of some thirty to fifty feet. Modulation
does not render the carrier audible to the ear. However, with the unmodulated carrier
directed toward the receiver, speaking into the carrier microphone produces audible
signals in the receiver audio output system. With the carrier removed, the audible
signal disappears.
Remarkably pertinent effects may be produced by reflecting a second carrier wave
path, in addition to the original path, from reproducer to microphone, as shown
in Fig. 7. Fading and phase distortion, such as encountered in standard radio
reception, may be produced realistically in the ultrasonic receiver by varying the
position of the reflector, the motion of the reflector being somewhat analogous
to the motion of the Heaviside layer. The movement of people within range of the
apparatus produces like results and indicates the potential utility of ultrasonics
for intrusion or passage detection in air mediums, using the beam-of-sound rather
than the beam-of-light principle.
In the near future it is proposed to conduct out-of-door tests in an effort to
empirically determine the range of such apparatus.
References
1. Lord Rayleigh, "Theory of Sound."
2.Dr. L. Bergmann, "Ultrasonics," P. 195.
3. Ibid. (2), P. 194.
Posted July 22, 2022 (updated from original post on
1/29/2015)
|