December 1950 Radio-Electronics
[Table of Contents]
Wax nostalgic about and learn from the history of early electronics.
See articles from Radio-Electronics,
published 1930-1988. All copyrights hereby acknowledged.
|
For some inexplicable
reason, the first page of Part 1 of the "Electric Space Ships" articles in
Radio-Electronics magazine is nowhere to be found. It is missing from the
scanned copy, so I am attempting to get a hard copy on eBay (December 1950 issue).
Until then, here is what is available from the PDF version on the WorldRadioHistory
website (I normally only use my own purchased hard copies). One RF Cafe visitor
wrote when I originally posted Part 2 that he has searched in vain for the
missing portion of Part 1 and thinks there might be some kind of conspiracy
to hide the information - part of the
ET/UFO cover-up. Maybe
the government has gone around and ripped page 32 from every issue of the magazine
;-) Otherwise, note in Figure 2 the "electric
wind" affecting the candle flame. This flame-related phenomenon is likely the
principle which Lee de Forest exploited in his
early
radio signal detectors which, eventually, led to his Audion invention. Here
is Electric Space
Ships Part 2.
Electric Space Ships: Part I - Using the sun's energy
Who Is Dr. Oberth?
Modern rocket research may be said to have two "fathers," the American Prof.
Robert H. Goddard and the German Prof.
Hermann Oberth. Chronologically
the priority belongs to Goddard; he was born in 1882. Oberth in 1894. Goddard published
his first work on rocket theory in 1919. Oberth in 1923. The important and significant
difference was that Goddard called his book A Method of Reaching Extreme Altitudes,
while Oberth boldly wrote The Rocket Into Interplanetary Space on his title
page. The two fathers" of modern rocket research are therefore, the fathers of cousins:
Goddard got the theory of rockets underway, Oberth the theory of space travel.
Having been associated with Oberth in his "early days" I find it difficult to
write just o short introduction about him. Mention of his name naturally invokes
countless memories. Of evenings spent at the shore of the Baltic Sea, looking for
the planets and talking about their surfaces. Of night walks through the Tiergarten
in Berlin, discussing long range rockets. Of discussions of moving under lunar gravity,
taking place in the shifting sands of the enormous moonscape which Fritz Lang had
built in the Uta studios in Neubabalsberg near Berlin for the film
The Girl
in the Moon, all copies of which were hastily coiled back later by the
Nazis when the research institute in Peenemünde was organized.
Whether you want to call Prof. Oberth a German, an Austrian or a Rumanian depends
entirely on the definition you have in mind. He was born in the city of Hermannstadt
in Transylvania on June 25, 1894. The people in that area constituted a German "colony"
much like our so-called Pennsylvania Dutch. Since his father was a physician it
seemed indicated that the son would be one too. But, at the University of Munich,
where he went to study medicine, he also studied physics and astronomy, gradually
with more and more emphasis an their mathematical aspects. When the first World
War broke out he was drafted into the Austrian army and finally assigned to the
Medical Corps. The periods of idleness to which every man in uniform is subjected
were the times when Oberth first thought about space ships and when the foundations
of space travel theory began to form in his brain.
After the war he wrote his book Die Rakete zu den Planetenraumen. Almost simultaneously
with its publication he got his first job: that of professor of mathematics in Mediash,
Transylvania. The book was a small one, as far as volume is concerned, not quite
a hundred pages of mostly mathematical type. Judging in retrospect over o quarter
century of time one can be sure that it will later be said that this book changed
human history, as profoundly as Columbus' trip across the ocean.
After having helped Fritz Lang with his movie and so ne experimental work on
rockets in Berlin in 1929-30, Oberth had to return to Transylvania since nobody
then saw any reason for spending money on rocket research. After the Nazis had taken
power in Germany Oberth was called to Germany and after several and long delays
was finally sent to the rocket research institute at Peenemünde, where the V-2 rocket
was created. But while Oberth found many of the principles he had first worked out
embodied in that rocket, he also found a virtually finished rocket on arrival, and
did not contribute directly to the V-2.
Herr Oberth is the Honorary President of the German Space Travel Society which
was re constituted after the war. He was the first to win the French REP-Hirsch
Prize before the war. He was the first man to be made an Honorary Fellow of the
British Interplanetary Society. And - most important of all - he was the first who
took the idea of space travel out of the realm of dreams and transferred it to the
department of science - Willy Ley.
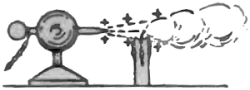
Fig. 2 - If the electric charge on the point is strong enough,
it creates a wind that will blow out the candle.
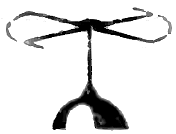
Fig. 3- An electric windmill - Charges leaking off the points
create a reaction force that makes the vanes of the mill rotate.
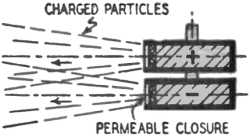
Fig. 4- Electrodes for the spaceship. Charged particles oozing
through the end are repelled by the charge within.
By Professor Hermann Oberth
...electrically charged particles for their part repel the point with the same
force with which they are in turn repelled by it. This is the basis of the electric
windmill (Fig. 3).
In denser air the electric wind blows slowly, though this is compensated by a
greater impulsive force or impact, compared with the energy used. In thinner air
its velocity is higher, but the impulsive force is less compared with the energy
produced. In denser air the electrically charged particles (charged molecules, ions,
or electrons) pass between the uncharged molecules of the medium and drag them along,
at the same time, of course, the particles' initially very rapid flight being thereby
slowed down. Accordingly, if there is a great deal of air around the charged particles,
a large mass of air is set in motion - but with only a small velocity; if the air
is thin, the charged particles encounter fewer uncharged molecules, and the velocity
of the gas streaming away is correspondingly higher. In a highly evacuated
Geissler tube, for example,
the molecules and atoms which fly from the anode develop mean velocities of 30 to
250 miles/sec, whereas in contrast to this the cathode emits free electrons for
the most part which, because of their small mass, can reach velocities of 56,000
miles/sec.
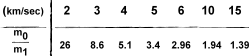
Table 1 - c and m0/m1 if v1-v0
= 6.5 km/sec
For a given quantity of energy supplied, the recoil action increases with the
increase in the quantity of matter repelled, but the velocity of repulsion diminishes.
This is also true in a vacuum where all the repelled particles are electrically
charged and the latter no longer drag along any uncharged molecules in their flight.
In this case, when there is very little matter repelled, the electricity carried
along will accumulate to form a high potential-causing a more rapid flight of the
particles. When more particles are present, one will soon come along which will
carry off the charge unit brought in, the potential will no longer rise as high,
and the particles will move slowly, but in compensation for this, the current strength
increases.
Furthermore, the recoil effect on an electric windmill is greater the denser
the air medium. In the case of denser air or lower potential, the particles which
are flung off nevertheless remain longer in the neighborhood of the rotating wheel,
and therefore have more time in which to exert their repulsive force on it.
Accordingly, as indicated in equation (1), it is possible with the same consumption
of material to develop a greater recoil momentum in highly rarefied gases, as a
result of the high recoil velocities (though at the cost of greater expenditure
of energy).
A perceptible electric wind arises only when enough electrically charged molecules
are set in motion. In dense air the electric wind can be produced only in the vicinity
of charged points where the charge density is very large, unless the potentials
are millions of volts. But if we are dealing with high rarefaction of the surrounding
gas, the electric density is sufficient to charge a suitable percentage of the surrounding
air molecules; in which case the electrodes need no longer be pointed, and the charged
particles are emitted from rounded or flattened surfaces and proceed in a direction
perpendicular to the surface.
If air or other gases are completely absent, such a stream of particles can be
generated only if the electrodes themselves supply the particles. This is the case,
for example, of the hot cathode which surrounds itself with a cloud of electrons,
of the potassium-containing photo cell which upon irradiation with ordinary light
emits charged particles, or of anodes which either liberate gases or which consist
of fused salts as in the
von Gehrke-Reichenheim method and from which individual ions are torn loose,
etc.
Electrodes
Accordingly, the electrodes of the electric spaceship might look like the model
in Fig. 4. This shows a cylinder whose walls and one end are impermeable to both
gas and electricity, but the other end of which is fitted with a permeable material.
This end can be either porous or at least permeable so far as the contents are concerned.
In the cylinder is a substance which, if the end piece is porous, had best be a
liquid, though it can also be a solid if its vapor tension is high enough (solid
carbonic acid or ice). This material, or at least its vapor, oozes through the porous
end of the cylinder. Since the cylinder is highly charged, the charged particles
fly off with considerable velocity.
The quantity of matter which trickles through the porous end of the cylindrical
electrodes depends on the nature of the porous material and on the chemical nature
and temperature of the contents of the cylinder. It would be most useful to provide
the electrode with an electric heating device, which would keep the temperature
at the desired level. Then the velocity of efflux and acceleration could be regulated.
Up to now everything pertaining to the invention of the electric spaceship is
quite clear. But in the second part of this article, I shall make several reservations.
Problems arise which can be solved only when enough measurements and data concerning
the nature of interplanetary space are available.
For example, the behavior of the electrodes of Fig. 4 can be predicted only when
the effect of various radiations which are absorbed by our atmosphere on the processes
taking place at the porous end of the electrode is known. It is therefore pointless
to introduce detailed calculations based only on the experience gained from Geissler
tubes.
One Possibility
This uncertainty is particularly large in the selection of the source of current.
It appears that a thermopile would be the simplest and most suitable device. Fig.
5 shows a piece of the thermopile on an enlarged scale and in a rather schematic
representation.
The dashed arrows indicate the incident rays of the sun. One junction of the
thermopile is turned to the sun and is also irradiated by reflected sunlight from
below: the other junction is in the shade. The sheet-metal strips can be soldered
together on the earth and wound on a drum in such a way that they are bent in the
proper position on the upper side, which can be done very easily mechanically. If
one wants to go further, one can either roughen the strips in those places shown
in black in the diagram or paint them black, and at the same time polish the places
shown in white. The effectiveness of the arrangement will be increased by this operation.
From measurements of the temperature of the surface of the moon it is possible to
calculate that the side turned to the sun will be heated to about 140° C., while
the side in the shadow will drop to a temperature of 100° C. below zero.
The potential difference between two adjacent metal strips is only of the order
of a hundredth of a volt, but when several hundred thousand of these are soldered
together in series, a marked difference of potential between the ends can be obtained.
These connected strips could be wound about the spaceship in a helical line, and,
since the acceleration of the spaceship is small, as has been brought out above,
it would hardly be necessary to reinforce or stiffen this helix.
The question now, however, is whether it will fulfill its function; for it is
possible that certain cosmic radiations might cause an equalization of the potential
difference between the metals at the ends of the thermopile, somewhat in the way
the electron cloud about a hot cathode tends to bring about the same result. It
is also possible that the traces of gases found in cosmic space might discharge
the electric charge built up in some other way than the desired one (though I do
not believe this will happen), and so on.
Ulinski,
the first to suggest such a thermopile (though his design was not very promising),
thought of providing it with an insulating coat. But an application of this sort
would have considerable weight and would tend to prevent the warming and cooling
of the proper junctions. Furthermore organic materials would soon be destroyed by
the cosmic radiations, an insulating coat of glass or some ceramic material would
easily crack and split off, because of the temperature difference and the corrective
bending of the metal helix, etc. I do not mean to imply that Ulinski's suggestion
cannot be realized, but simply wish to indicate that many questions regarding it
remain unanswered, and that the final result might very well be far less promising
than it appeared at first sight.
In Part 2
of this article we shall consider the detailed description of a feasible spaceship
and its powerplant.
1. Other figures are frequently given for both the distance of the orbit
above the earth and for the velocity of rotation. This is due to the fact that the
authors concerned have failed to take into account the fact that this station would
not travel about the earth in one solar day of 24 hours. but in a sidereal day,
if it is to remain over the same meridian.
Mathematical Analysis
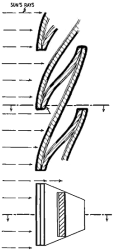
Fig. 5 - Series-connected thermopiles have been proposed as a
method of collecting energy to drive the spaceship.
The principles of rocket propulsion are interesting to contemplate.
If the mass of the material expelled each second with a velocity cl is m1, then
the recoil momentum is measured by:
P1 = m1c1
(1)
However, the total energy which must be expended on this propellant in each second
to set the mass mi in motion with the velocity cl, to get this recoil effect, is:
E1 = ½m1c12
(2)
If we consider another mass m2 with the velocity co, then the corresponding equations
are:
P2 = m2c2
(3) E2 = ½m2c22
(4)
If the source of energy does the same work in both cases, then
E1 = E2
and from equations 2 and 4 we have:
m1c12 = m2c22
(5)
If we now eliminate m1 from equations 1 and 5, then divide the result by equation
3 we get:
P1:P2 = c2:c1
(6)
This means that for equal work performed by the source of energy, the recoil
effect is inversely proportional to the velocity of efflux. That is, the smaller
the velocity of efflux, the greater the recoil itself.
But it follows from equation 5 that the loss of material depends on the square
of the velocity of efflux. This means, in agreement with equation 1, that the mass
of propellant is consumed more sparingly the higher the velocity of efflux, but
this is at the cost of greater energy consumption.
For example, if the electric spaceship expels 10 tons of matter at the rate of
10 km /sec and during this time it reaches a velocity of 2 km /sec, it would be
able to expel only 2.5 tons of propellant and reach a velocity of only 1 km /sec
with a velocity of expulsion of 20 km /sec. But if it worked four times as long,
it would again consume the original mass of 10 tons, but would then reach a velocity
of 4 km /sec.
We are dealing here with an inverse relationship. For a given amount of energy,
the more rapidly the mass is expelled, the smaller is the amount which can be expelled
in unit time, and the slighter is the recoil and hence the acceleration. But, using
the same quantity of propellant, the spaceship can continue to accelerate for a
longer period of time. This longer period of time has the same ratio to the shorter
period as the square of the higher velocity of efflux has to the lower velocity
of efflux. Therefore the spaceship reaches a velocity at the end of the longer period
of acceleration which has the same ratio to the velocity reached in the shorter
period as the higher velocity of efflux has to the lower.
The electric spaceship is certainly not very exacting as to the fuel it would
use. Anything might serve as propellant if it will diffuse readily through the porous
end of the cylinder and if it is sufficiently inert so it will not attack the walls
of the electrodes.
Posted May 26, 2021
|