One of the photos in this
1958 Radio & TV News magazine article on the Jodrell Bank radio
telescope shows what appears to be the largest
multi-conductor cable connector I have ever seen. It looks like a early
Photoshopping of a DB-9 connector with a heavy metal back shell. The cable
bundle is three to four inches in diameter. Rather than use slip rings to
transfer the control, data, and power signals from the base to the steerable
250-foot diameter parabolic dish of the Jodrell Bank radio telescope (now called
the
Lovell telescope), a single massive cable does the job. The science of radio
astronomy was barely three decades old at the time it was built. It was in 1931
that Karl Jansky
first determined that radio signals were coming from our Milky Way galaxy. He
eventually ended up working for Bell Labs in Homdel, New Jersey, where he built
a radio telescope to investigate background noise in the 10-20 meter wavelength
band, where Bell planned to use its microwave relay system.
Related articles: "How
We Listen to Stars and Satellites" - January 1958 Popular
Electronics, "Radio Astronomy and the Jodrell Bank Radio Telescope"
-
February 1958 Radio & TV News, "Radio Astronomy - Low
Noise Front-Ends" - June 1954 Radio & Television News, "Radar
Explores the Moon" - May 1961 Popular Electronics, "Cosmic
Radio Signals from Sun and Stars" - March 1948 Radio-Craft
Radio Astronomy and the Jodrell Bank Radio Telescope
By A. C. B. Lovell
Director, Jodrell Bank Experimental Station
The complete story about the powerful new tool that has been making headlines
tracking the Soviet satellite.
The great steerable radio telescope which has been built at Jodrell Bank in Cheshire,
England, for the University of Manchester has recently been put in operation. It
is to be used with two distinct types of radio equipment for the exploration of
the universe: first, as a receiver to pick up the radio waves which are generated
in the remote parts of the universe, and second as a combined transmitter and receiver
for investigations within the solar system using the radio echo or radar technique.
It was this latter that was recently made such good use of in the tracking of the
Soviet earth satellites that were launched a few months ago.
First of all, however, it may be useful to say a few words about the fundamental
reasons which led to the idea of such a huge and expensive instrument.
The exciting discoveries in astronomy have depended mainly on the development
of optical telescopes of ever-increasing size. These large telescopes require unusual
climatic conditions if their potentialities are to be realized; the great telescopes
of the twentieth century have been built in America at Mt. Wilson and Palomar.

Giant 250-foot radio telescope, standing amid Cheshire farmland,
is tilted for recent tests.
The 100-inch telescope on Mt. Wilson came into operation just after the First
World War and, in the hands of the American astronomers, it revealed a universe
of unforeseen immensity. Hitherto, the assemblage of stars was believed to be contained
in a relatively small volume some few thousand light years in extent, with the sun
and planets near the center. The many nebulae to be seen in the telescopes were
thought to be diffuse objects lying within this system. But this new telescope revealed
that the Milky Way system, or local galaxy, was disc-like in structure, extending
for 100,000 light years across its plane and containing about 10,000 million stars.
Moreover, Hubble's investigations with the telescope soon convinced his colleagues
that many of the nebulae were external to this system, and that the nearest one
in Andromeda must be separated from the local star system by over a million light
years, and must also contain a vast number of stars. Other nebulae were shown to
be similar star systems at even greater distances. Nowadays, it is known that the
200-inch telescope on Mt. Palomar can see nebulae out to distances of hundreds of
millions of light years, and within the field of view there must lie an enormous
number of galaxies - perhaps of the order of a thousand or ten-thousand million.
Origin of Radio Astronomy
In the face of this penetration to the remote depths of space it seemed hardly
possible a few years ago that the remaining tasks of observational astronomy could
be other than the detailed study of the individual stars and nebulae as seen in
the conventional telescopes. But by a strange twist of fate the Second World War
was to place a new and enormously powerful tool in the hands of astronomers for
the exploration of space, and the recent discoveries have rivaled in excitement
those made in the last twenty years with the ordinary telescopes.
Editor's Note: We are proud to welcome to these
pages one of the world's leading authorities in the new science of radio astronomy,
A. C. B. Lovell, O.B.E., F.R.S. is Professor of Radio Astronomy at the University
at Manchester in England and has been Director of Jodrell Bank Experimental Station
since 1951. Born in 1913, Lovell attended school in Bristol as well as the University
of Bristol. He began his teaching career in 1936 at the University of Manchester,
worked at the Telecommunications Research Establishment from 1939 to 1945, and has
since been teaching at the University of Manchester. In 1946, he was awarded the
Order of the British Empire and in 1955 was made Honorary Foreign Member of the
American Academy of Arts and Sciences and Fellow of the Royal Society. Among the
books he has written are "World Power Resources and Social Development," "Radio
Astronomy," and "Meteor Astronomy." Many of his articles have been published in
leading physical and astronomical journals.
Almost all astronomical research has been carried out with telescopes and other
instruments receiving light waves emitted by the stars in the visual part of the
electromagnetic spectrum. Various auxiliary instruments such as photoelectric cells
have been used to extend the studies a little beyond the visual limits into the
infrared and ultraviolet regions, but appreciable extension is prohibited by the
absorption caused by water vapor and fine dust in the earth's atmosphere. Thus,
our knowledge of the universe has arisen almost entirely from the study of stellar
emissions in this narrow visual and near-visual region of the spectrum.
It was, therefore, surprising when an American engineer, Jansky, discovered at
the end of 1931 that radio waves, apparently emanating from regions beyond the solar
system, were reaching the earth. Jansky's work caused little immediate interest
and the only important additions to his results before the Second World War were
made by Grote Reber, an amateur investigator who constructed the first radio telescope
of the type with which we are familiar today. It was 30 feet in diameter and received
radio waves on a wavelength of about two meters. The instrument could readily be
pointed to different parts of the sky and with it Reber confirmed Jansky's discovery
that radio waves were reaching the earth from outer space.
He found that the radio signals were strongest from directions near the center
of the local galaxy and along the plane of the Milky Way, in fact, that the radio
signals were roughly proportional in strength to the concentration of stars in the
direction in which the radio telescope was pointing. On the other hand Reber failed
completely to detect any signals from the bright stars or from other prominent features
visible in telescopes.
Even today, after many years of rapid technical development, only a very few
objects identifiable in the large telescopes are known to be the source of some
of these radio emissions. Apart from the sun, no one has yet found radio emission
from any common type of star. On the other hand, the rare supernovae, or the gaseous
remains of exploded stars in the Milky Way, are known to be radio emitters. Probably
most of the radio waves in the Milky Way come from such types of object together
with that generated in the interstellar gas which fills the space between the stars.
The reason why most of the radio sources, or radio stars, have not been identified
is now believed to arise from their very great distances which put them beyond the
range of even the largest optical telescopes. For example, the second most powerful
radio source in the sky lies in the constellation of Cygnus, and this has been identified
as the collision of two entire galaxies of stars at a distance of 200 million light
years. This is about the useful limit of penetration of the 200-inch optical telescope
on Mt. Palomar, and it seems possible that many of the weaker radio sources may
be similar cases of galaxies in collision at even greater distances. We shall return
to this when discussing the uses of the new radio telescope.
New Radio Telescope
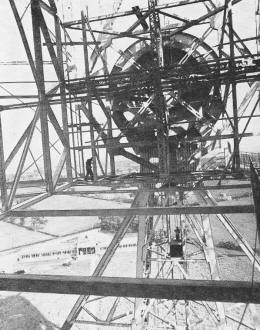
Here is a view of one of the great trunnions on which the giant
reflector bowl turns with the control building far below.
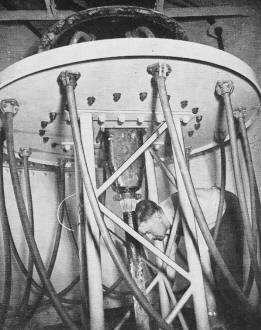
The method by which the cables are brought on to the moving portion
of the radio telescope. Slip rings are not used.
The study of the radio emissions from space is carried out on a wavelength a
million times longer than the wavelength of the light waves which are focused by
the conventional optical telescopes. The radio waves are unaffected by cloud, fog,
or daylight and, in this respect, the radio astronomer has a marked advantage over
the traditional methods of astronomical investigation. On the other hand, because
of the long wavelength, it is extremely difficult to achieve any appreciable resolution.
The beam width, or the angle of the cone in which the radiation is received, depends
on the ratio of the wavelength to the diameter of the telescope. Thus to achieve
the same resolution as a very small optical telescope, the aerials of a radio telescope
would have to extend for thousands of miles. The need for the maximum possible resolution
in the radio work has been a dominant feature of the technical developments. A great
deal has been achieved by special devices in which two similar aerial systems, spaced
by several hundred yards, are connected to common recording equipment. This type
of radio telescope, known as an interferometer, has been developed in Cambridge
(England) and Sydney.
In an alternative approach, the physical size of the aerial system is increased.
There are now several steerable radio telescopes of small size in existence. Many
years ago a large radio telescope with an aperture of 220 feet was constructed at
Jodrell Bank, but this was fixed to the earth and could be used only to explore
a small part of the heavens. Experience with this instrument soon demonstrated the
vital role which could be played by a completely steerable telescope of this order
of size. The engineering difficulties and expense of such an undertaking are formidable.
Nevertheless, the results to be anticipated were such as to enlist the sympathetic
interest of many prominent scientists when the idea was first put forward in 1949.
Telescope Crucial Instrument: In conventional astronomical investigations, the
telescope has been the crucial instrument for the exploration of space. Successive
increases in size have led to more light-gathering power and greater resolution,
and although the improvements in auxiliary instruments, such as photographic plates
and spectroscopes, have been very important, nevertheless the great advances in
observational astronomy have come primarily from larger and larger telescopes. The
situation in radio observations is very similar: whereas large optical telescopes
are required to improve the light-gathering power and the resolution, large radio
telescopes are required in order to be able to pick up faint signals at greater
distances from the earth as well as for greater resolution.
The new telescope is essentially a paraboloidal steel bowl 250 feet in diameter,
with the focus in the aperture plane, built so that it can be directed towards any
part of the sky. In principle the motion of the telescope is altazimuth. The bowl,
which weighs about 700 tons, is driven in elevation by a Ward-Leonard system through
two 27-foot racks from the dismantled battleship "Royal Sovereign. These are mounted
170 feet above ground on two towers which themselves rotate on a 350-foot railway
track to give the motion in azimuth. The drive is via two railway trucks under each
tower, again through a Ward-Leonard system. Four additional trucks, which are undriven,
serve as wind carriages on each side of the structure. The towers are connected
near ground-level through a heavy diametral girder system supported on the central
pivot, which is the fundamental locating point of the telescope. The power and instrumental
cables come through this central pivot into a motor room situated within the diametral
girder immediately above the central pivot. This room contains the motor generator
sets and controls for the Ward-Leonard system that is employed.
17-Foot Gauge Double Track: The 17-foot gauge double railway track on which the
telescope rotates is mounted on deep-piled foundations which extend, in some places,
90 feet underground. The various power, control, and instrumental cables are taken
into an annular laboratory underneath the central pivot and then through an underground
tunnel to the control room. This control room houses the main control racks and
console. The computer system consists of magslip resolvers working in servo loops
to solve the necessary equations in order that the telescope can be given a side-real
movement. A wide range of movements can be selected at the control desk; for example,
automatic sidereal motion at a given right ascension and declination, motion in
galactic latitude and longitude, straightforward motion in azimuth and elevation,
and various automatic scanning movements with a choice of rasters. Parallax corrections
can also be introduced when it is desired to track a body in the solar system. There
are no slip rings so that the danger of creating electrical interference is avoided,
and the limit of motion is 420 degrees, after which an automatic reversal takes
place.
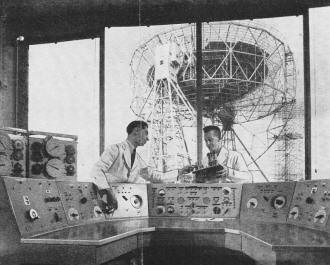
Here is a view of the Jodrell Bank radio telescope which has
been taken from the operating console at which the controller sits. During the night
the great telescope is illuminated by floodlights in order that the controller may
be able to keep everything in view and monitor the position of the immense bowl.
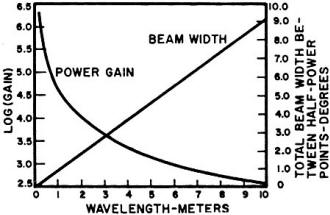
Fig. 1 - Calculated power gain and beam width of the 250-foot
aperture radio dish.
The specification calls for a tracking accuracy of at least 12 minutes of arc
at speeds up to 4 degrees per minute. The maximum slewing speed is about 22 degrees
per minute in azimuth and elevation. The position of the telescope in azimuth and
elevation is repeated back to the control room through magslips driven by accurately
machined chain racks independently of the driving system. The specification requires
these positions to be repeated to an accuracy of ±1 minute of arc.
Reflecting Membrane 1/12" Thick Steel: The reflecting membrane is of 1/12"-inch
thick steel sheet welded from 7000 individual sections of about 3 ft. x 3 ft. onto
the purlins of the steel framework. It has been essential to ensure good conductivity
across these welded sections, otherwise the membrane would become very "lossy" at
certain wavelengths. The primary aerial feed is carried at the focus on a steel
tower built up 62 1/2 feet from the apex of the paraboloid. This tower diminishes
in cross-section rapidly with height in order to avoid obscuration and scattering
from the primary feed. It was, however, essential to design it with sufficient stiffness
so that no displacement occurred as the bowl turns over. An important scientific
requirement is that easy access can be obtained to the primary feed so that the
operational wavelength can be changed readily. Originally it was intended to have
an access tower which could move out on tracks on top of the diametral girder to
put the operator in reach of the aerial when the bowl was inverted. This was later
abandoned in favor of a system whereby the aerial is mounted on a 50-foot steel
tube which slides into the top of the aerial tower. With the bowl inverted this
can be winched down to ground-level and replaced by another 50-foot tube complete
with aerial system. The radio-frequency cables from the aerial run inside this tube
and can be reached from a small platform near the base of the tower when the bowl
is facing towards the zenith.
In much of the work it will be necessary to mount the radio-frequency preamplifiers
and other parts of the receiving equipment as close as possible to the aerial. These
essential units will be contained in a small laboratory which swings underneath
the bowl. Further laboratory space is available at the tops of the two towers, but
even from those the minimum length of cable run to the primary feed is about 200
feet. Other scientific apparatus will be installed on the base girders; but the
main recording apparatus will be in laboratories that are situated adjacent to the
control room.
The theoretical curves showing the beam width and power gain as a function of
wavelength are given in Fig. 1. Calculations have been made of the effect of irregularities
in the membrane on the power gain, from which it is clear that, except at very short
wavelengths for which the telescope is not primarily designed, large distortions
are permissible without any appreciable effect on the performance of the telescope.
During the next few months it is hoped to begin the scientific program by establishing
these curves on an experimental basis by observing certain radio sources at different
azimuths and elevations over a range of wavelengths.
Immediate Tasks
A common feature of the surveys of radio stars in both northern and southern
hemispheres is the failure to associate all but a few with celestial objects visible
in the large telescopes. For example, a recent Cambridge survey located 1936 radio
stars, 1906 of which were of small angular diameter and distributed isotropically.
Only a few per-cent of these have been successfully associated with known objects
and the surveys in the southern hemisphere by the Sydney group give similar results.
The spatial distribution of these unidentified radio stars has been investigated
by plotting log N against log I where N is the number of radio sources per unit
solid angle with an intensity greater than I. If the sources are distributed uniformly
throughout space it is easy to show that the plot should give a straight line of
slope -1.5, so that any departure from this relationship will give information about
the variation of spatial density with distance. The results of the log N/log I Cambridge
plots are very surprising. There is a marked departure from the expected slope,
the experimental curve becoming steeper for low intensities until a flattening due
to instrumental limitations occurs. The implication is that the spatial density
of radio stars is constant in the neighborhood of the solar system but progressively
increases with distance. It is impossible to account for this result and for the
isotropy of the sources other than by assuming that the increase in slope is taking
place at distances which are comparable with the limits of observation of the 200-inch
telescope. If, in fact, this spatial distribution is due to processes on a cosmical
scale, then the results have a significant bearing on cosmological theories. In
the steady-state or continuous-creation theories the density of nebulae should be
everywhere the same and independent of time and space, since new galaxies are continually
being formed to take the place of those which move out of the field of view as a
result of the expansion of the universe. On the other hand, in the evolutionary
theories the spatial density decreases progressively with time. In this case we
should expect to find a greater concentration of nebulae near the limits of the
observable universe which corresponds to a period of time some 2000 million years
ago.
Lead to Further Conclusions: This is precisely the result which appears in the
radio star distribution, but since the radio emission from individual nebula is
too weak to be detected at such distances we are led to further conclusions about
the nature of these radio sources. Galactic collisions of the Cygnus type can be
shown to produce radio emission of the order of magnitude required, and the suggestion
is that these sources are colliding galaxies probably lying beyond the limit of
penetration of the 200-inch telescope. This is consistent with the interpretation
of the log N/log I curve, since at the time of several thousand million years ago
both the spatial density and the number of colliding galaxies must have been much
greater than at present.
This interpretation of the log N/log I curve for the observed radio sources is
therefore in favor of the evolutionary cosmological theories. This conclusion is,
at present, in very great dispute, particularly as the preliminary results of the
Sydney survey do not entirely agree with those obtained in Cambridge. The issue
is of the utmost importance, carrying with it the possibility of a significant contribution
to the main cosmological problem of the origin of the universe. Before agreed conclusions
can be reached, the validity of the data must be greatly increased, and this program
can be expected to form a very prominent aspect of the work of the new telescope.
In the program as planned now, the telescope will be used on a frequency of 168
mc. as an element in an interferometer to measure the actual angular extent of the
sources so that the effective temperatures of the individual sources can readily
be determined.
Additional Uses: The foregoing is an example of the use of the new telescope
in programs where it will be used as a receiving aerial. In addition to this cosmological
study on 168 mc. the telescope is equipped with receiving systems on 90, 408, and
1420 mc. for surveys of the distribution of radio emission from the local galactic
system and for the investigation of the strength of the radio waves emitted by various
types of normal extragalactic nebulae.
In addition to this type of work the telescope will be used as a combined transmitting
and receiving aerial. In these radar or radio-echo aspects of the work, the moon,
artificial satellites, and the planets will certainly figure prominently.
The moon echo experiments show that the radio echoes are subject to deep and
rapid fading - an effect which is now believed to be due to a peculiarity of the
moon's motion with respect to the earth, known as libration. The lunar echo apparatus
at Jodrell Bank works on a frequency of 120 mc. and uses a transmitter giving 10
kw. in 30 millisecond pulses at a recurrence rate of 0.6 per second. The receiver
bandwidth is 30 cps, and appropriate arrangements have to be made to allow for the
Doppler shift in the frequency of the returned signal. The most important results
obtained with this apparatus concern the long-period fading (20 to 30 minutes),
which by cross-polarization experiments has been shown to be due to the rotation
of the plane of polarization of the radio wave as it traverses the ionosphere (the
Faraday effect). This immediately led to the development of a moon echo system by
which the total electron content of the ionosphere could be determined.
The magneto-ionic theory shows that the polarization shift depends on the total
electron content of the ionosphere per square centimeter along the line of sight.
Experiments on a single frequency can give only the rate of change of the electron
content, and it is necessary to carry out the measurements on two closely spaced
radio frequencies. Measurements of the total electron content by this means indicate
that the electron content is considerably greater than that estimated on the basis
of a simple parabolic region.
Telescope Removes Handicaps: The technical difficulties in this work are considerable,
and with the present aerial system, measurements can be made only with the moon
in transit for about 10 periods in each lunation. The new telescope will immediately
remove these handicaps and will enable systematic data to be collected about the
total ionospheric electron content. This is bound to be of considerable importance
to our understanding of the ionosphere and of solar terrestrial relationships.
The problem of radio echoes from the planets is vastly more difficult, and, as
far as is known at present, no serious attempts have yet been made to solve it.
Success in detecting radio echoes from Venus would demand an over-all power sensitivity
between a million and ten million times greater than that required in the case of
the moon. This assumes, of course, that the reflection coefficient of the planet
would not be inferior to that of the moon. The problem cannot be appreciably eased
by increasing the length of the transmitter pulse with appropriate decrease of receiver
bandwidth, because of the Doppler spread introduced by the rotation of the planet.
The rotation period of Venus is unknown (this would, in fact, be one of the main
scientific results to be expected from the experiment), but on the basis of current
estimates of the rotation period, the Doppler spread would probably limit the useful
pulse width to about 40 milliseconds, which is only somewhat longer than that used
in the lunar investigations. The main factor must therefore be achieved in the gain
of the aerial, by increasing the transmitter power, and possibly by integration
of successive echoes.
Attempt to Secure Planetary Echoes: This problem has been carefully considered
at Jodrell Bank in relation to the very great gain of the new telescope, and an
attempt to obtain planetary echoes will be made early in the research schedule.
The complete return journey of the earth-Venus radio signal will take 4 minutes,
and success in detecting such a radio echo would be a spectacular technical accomplishment.
Nevertheless, the experiment could not be justified on this basis, and it is hoped
that with the telescope a systematic program will be possible, in which the rotation
period can be determined and information obtained about the Venusian surface and
atmosphere.
A further interest in this planetary work is the possibility of measuring the
range of the planet with sufficient accuracy to improve our knowledge of the solar
parallax. The present position is that the two most accurate optical measurements
should be correct to 1 part in 10,000 but differ between themselves by 1 part in
1000. The possibility of improving this measurement by the radio echo technique
will certainly receive immediate attention if the initial attempts at detection
are successful.
The foregoing constitute a few examples of the type of work on which the telescope
is likely to be engaged during the next few years. Its field of activity is enormous
and as opportunity allows it will be used to study the radio emissions from the
sun and planets in addition to the galactic and extragalactic work already mentioned.
On the transmitting side, in addition to the lunar and planetary work it will be
deployed in the study of radio echoes from very faint meteors, from the aurora borealis,
and from the earth satellites. The telescope enters on its research career at a
moment of extreme interest, and it may well be expected to make a significant contribution
to astronomy and various aspects of geophysics for many years to come.
Posted January 23, 2020
|