November 1960 Electronics World
Table
of Contents
Wax nostalgic about and learn from the history of early electronics. See articles
from
Electronics World, published May 1959
- December 1971. All copyrights hereby acknowledged.
|
When really good researchers
set out to write books on history, they do not simply cull information from the
publications of fellow contemporary authors; instead, they look for sources that
were published during or around the time of the subject being covered. Doing so
helps minimize the possibility that inaccuracies have crept into the knowledge pool
and that information other authors might have either deemed insignificant or have
missed can be recovered. With a bit of luck, sources are discovered that have never
been used before. That is part of my motivation for going to the trouble of buying
these vintage magazines and posting articles like this one which reports on early
maser developments. It delves fairly deeply into the solid state physics of rare
earth minerals that some of the first masers and lasers relied upon to function,
including energy band diagrams and cryogenics.
If the "sugar scoop" antenna looks familiar, it might be due to its rising to
fame as the result of Dr.s Arno
Penzias and
Robert Wilson having serendipitously discovered the cosmic background radiation
(CBR)
of the universe's creation while using it after Project Echo shut down. The discovery
led to a
Nobel Prize in Physics in 1978.
Articles here on RF Cafe which mention the Dr. Robert W. Wilson and Dr. Arno
Penzias are The
Maser & Sugar Scoop Antenna: Receiver for Signals from Space,
Bell Telephone Laboratories Project Echo,
The Amazing Maser: The Jewel That Conquers Space,
Cosmic Radio Signals from Sun and Stars, and
Sugar-Scoop Antenna.
The Maser: Receiver for Signals from Space
By Martin I. Grace & Joseph G. Smith
Airborne Instruments Laboratory, Div. of Cutler-Hammer Inc.
Radar pulses bounced back from distant planets, communications with space vehicles,
receivers to listen for radio signals from outer space, satellite-reflected telephone
and TV microwave signals - these are all possible because of the remarkable maser,
which uses atomic forces within a super-cooled ruby to amplify.
Some two-hundred times better than a good, conventional radar receiver, the maser
receiver affords scientists in the fields of space communications, radar, and radio
astronomy the possibility of amazing improvements. This is because the maser receiver
is an almost theoretically perfect receiver insofar as sensitivity and low noise
are concerned. Radar range can be increased, or for the same range, the transmitter
power can be cut by a factor of 10. Satellite and deep space communication will
be extended. Coast-to-coast and continent-to-continent microwave communication links
without repeater stations (i.e., via satellite reflector) should be realized. Radio
astronomers will be able to "see" much deeper into space, helping to answer some
of the basic questions about the universe, and perhaps, discover another civilization.
This is not as preposterous as it sounds. Project "Ozma" has been initiated to listen
continuously for intelligent transmissions of radio signals from outer space. The
project will use a maser for the ultimate in listening range.

Dr. R. H. Kingston prepares the maser for the Venus observations
at M.I.T. Lincoln Laboratory's Millstone Hill Radar Observatory.

Lincoln Laboratory's Radar Observatory at Westford, Massachusetts.
Venus was "seen" with the maser-equipped dish at the right. Frequency used was about
300-500 mc.; pulse power was 265 kw.; dish size was 84 feet. Maser is at feed point
of dish antenna.

Close-up of Harvard maser mounted at antenna feed. Protective
covers have been removed to show electromagnet at bottom and dewar structure that
is utilized. The receiving waveguide horn is located at left.
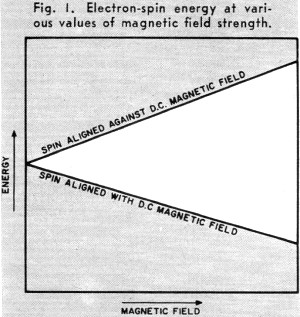
Fig. 1 - Electron-spin energy at various values of magnetic
field strength.

Harvard University's 60-foot parabolic dish with maser installed
at feed point.
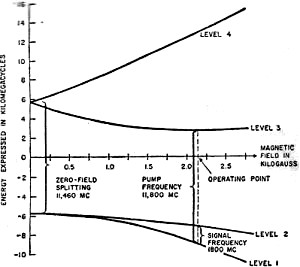
Fig. 2 - Energy-level diagram for ruby showing magnetic
field and pump frequency needed to operate at 1800 mc.

H. E. D. Scovil of Bell Laboratories points out to R. W. DeGrasse
an input coax of the two-channel traveling-wave maser designed for satellite communication.
This maser is being used with a horn-reflector antenna.

Fig. 3 - Energy-level spin population.
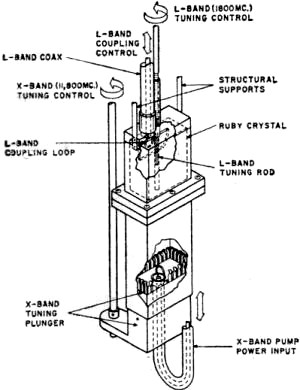
Fig. 4 - Tunable maser cavity structure used in Airborne
Instruments Laboratories gear.

Fig. 5 - System diagram of maser operation.

Fig. 6 - Comb-type slow-wave structure designed for traveling-wave
maser of type employed in Bell Telephone Laboratories and Airborne Instruments Labs.
equipment.
An excellent example demonstrating the ability of a maser to amplify very weak
signals was the Venus radar bounce disclosed last year. In this experiment conducted
at the Millstone Radar Site of the MIT Lincoln Laboratory, a radar signal was beamed
at the planet Venus. A small portion was reflected back toward earth where it was
detected by a maser receiver. The scientists involved in the bounce admit the project
would have been unsuccessful without the maser. Calculations showed that the transmitter
power necessary for the bounce, using a conventional receiver, would have been so
high that the air directly in front of the antenna would have been ionized.
Prof. C. H. Townes of Columbia University coined the word "maser" by taking the
first letters of its more technical description, "microwave amplification by stimulated
emission of radiation." Prof. Townes proposed a maser in 1953 and experimentally
verified it three years later with a maser which was essentially an ultra-stable-microwave
type oscillator. Based on these results, Prof. N. Bloembergen of Harvard University
proposed in 1956 a maser capable of continuous-wave amplification and, within three
months the theory was embodied in an operating unit.
How It Works
The maser is a rather exotic piece of equipment, combining a rare gem (usually
ruby) and extremely low temperatures to produce amplification. Unlike most other
devices in electronics, the maser makes use of bound electrons to accomplish amplification.
There is no flow of electrons as in a tube or transistor. It is a property of these
bound electrons that they spin on their axes very much the same way as the earth
spins on its axis. We all know that a moving charge is a current and, associated
with every current is a magnetic field. The spinning electron is charge in motion
and this causes the electron to have a magnetic field. Normally the spin is randomly
oriented but when this "electron spin" is placed in a d.c. magnetic field, the electron's
magnetic field normally aligns itself parallel to the d.c. field. The action is
similar to that of a compass needle.
The electron spin with its field aligned with the d.c. field possesses a discrete
amount of energy. Now, the electron spin can take up another position, namely, against
the field. Energy must be expended in "flipping" the spin over. Thus, an electron
spin aligned against the d.c. field has more energy than one aligned with it. In
a substance containing many of these spins, the condition pictured in Fig. 1
will exist. Some spins will be aligned with and some against the d.c. field. It
can be seen that two definite energy levels exist and that the difference in energy
is directly proportional to the applied d.c. magnetic field.
The above condition is for a substance possessing one net electron spin per atom.
If the substance possesses two net electron spins per atom, it will have three energy
levels - one for both spins aligned with the d.c. field, one for both spins aligned
against, and one for one spin aligned with and one spin aligned against the d.c.
field. In the case of three net electron spins, there are four energy levels and
so on. Chromium-doped ruby, which is the primary maser material today, has three
net electron spins per atom. Ruby is a single crystal of aluminum oxide with a small
percentage (.05%) of chromium. This small percentage of chromium is what gives ruby
its characteristic red color. It is also the chromium which provides the three net
electron spins necessary for maser action.
Quantum physics states that the difference in energy (Δ E) between two
levels can be equated to frequency (f) by the simple formula: Δ E = hf, where
h is Planck's constant. Now we can change the graph of energy levels and write frequency
in place of energy. Fig, 2 shows the energy levels for ruby plotted in this manner.
There are approximately 7 x 1010 electron spins in a cubic centimeter
of ruby and, of these, a certain percentage of spins are in each of the four energy
levels. The number of spins in each level a function of the temperature of the crystal.
At room temperature the four levels are almost equally populated but at liquid helium
temperature, 4.2° above absolute zero. the populations of the four levels are
substantially unbalanced. (Absolute zero is also known as zero degree Kelvin, or
K, and represents a temperature of 273 degrees below zero C or 460 degrees below
zero F.) The solid bars in Fig. 3 represent a typical energy population distribution
for ruby. From the diagram it can be seen that the lower energy levels are more
heavily populated than the higher ones.
The operation of a 3-level maser can be explained from an energy level diagram.
The term "3-level maser" means that only three energy levels are used in attaining
maser operation even though there may be a greater number of energy levels in the
material. If we inject into the crystal a strong r.f. signal from a local oscillator,
equal in frequency to the difference between energy levels 1 and 3, an interesting
phenomenon takes place. Some of the electron spins in the lower level absorb energy
from the r.f. field and jump to level three. If the local-oscillator r.f. signal,
usually called the "pump signal," is strong enough, the "pump" transition (level
1 to level 3) can be saturated, i.e., the number of spins in level 3 can be made
equal to that in level 1. The total number of spins in the two levels has not changed,
but now they are equally populated. Levels 2 and 4 are unaffected since their natural
frequencies have not been involved. This "pumped" condition is shown in Fig. 3
by the dashed bars. It can be seen that level 2 now possesses more electron spins
than level 1. This is contrary to the normal equilibrium condition.
If a signal which is equal in frequency to the difference between energy levels
1 and 2 is now fed into the crystal, spins in level 2 will be stimulated to emit
energy to the signal rather than absorb energy from it. In the process they will
flip over and fall back down to level 1. This giving up of energy to the signal
frequency is amplification. By varying the d.c. magnetic field, the operating point
in Fig. 2 is varied, thereby changing the spacing of the levels and "maser
action" can be obtained at other frequencies. This is the basic scheme of maser
operation. Sophisticated techniques have been developed using more than three levels,
double-pumping arrangements, and harmonic and sub-harmonic schemes; but it all boils
down to the same fact. To accomplish amplification, a greater population must exist
in a higher energy level than in a lower one.
The low-noise characteristic of a maser is more difficult to explain but it is
extremely important. In conventional receivers the main source of background noise
is from random emission from hot cathodes, shot noise in tubes, and random thermal
noise in resistors. Since, with the maser, amplification occurs without the use
of hot cathodes and tubes, it is logical to expect that noise from such sources
does not exist. Also, the components that could produce noise are at an extremely
low temperature, a few degrees above the point where all thermal motion ceases to
exist. This is only part of the low-noise story because, for quantum-mechanical
reasons, noise is even lower than the helium-bath temperature would predict.
Types of Masers
There are two basic types of maser configuration, the cavity maser and the traveling-wave
maser, or TWM.
The first maser amplifiers constructed were cavity masers. In this type, resonant
circuits are used to inject the r.f. signals into the ruby crystal. The early cavity
masers consisted of a single microwave waveguide cavity resonant at two frequencies;
the pump and the signal. The maser material was placed inside the microwave cavity.
This first type of cavity maser had the disadvantage of operating at a single fixed
frequency. Later, cavity masers of a tunable nature were designed. A tunable maser
structure is shown in Fig. 4. It consists of a waveguide resonant cavity which
is resonant at the pump frequency, and a quarter-wave coaxial resonator, resonant
at the signal frequency. The coaxial resonator is constructed inside the waveguide
resonator. The maser material is placed inside the resonant cavity. The r.f. signals
are coupled into the cavity by adjustable loops.
The cavity maser is a one-port amplifier; that is, the input and output have
the same common terminals. In order for this type of amplifier to operate, a non-reciprocal
device called a "circulator" is necessary. The circulator acts as a traffic cop
(see Fig. 5). A signal that enters port 1 is directed to port 2, a signal that
enters port 2 is directed to port 3; and so on around the loop.
The cavity maser has many inherent problems: (1) it must be manually tuned; (2)
the noise figure is degraded by "lossy" input components; (3) the unit's stability
is not too good; and (4) saturation effects occur at relatively low power levels.
At the onset of saturation the maser loses gain and becomes transparent, acting
very much like a piece of transmission line. The receiver's second stage is usually
capable of picking up the saturating signal and completing the reception. Once the
maser saturates, it takes a considerable amount of time after the saturating signal
is withdrawn to return to normal operation. If, during this time, a low-level signal
enters the system, it is lost. This is the only drawback of the maser. Presently,
this "recovery" time is on the order of 50 milliseconds but crystal-line materials
are being developed which will reduce this figure to insignificance.
The traveling-wave maser corrected most of the drawbacks of the cavity type.
It employs a transmission type of coupling between the r.f. signal energy and the
crystal rather than the resonant technique used in the cavity maser. There are many
advantages in this type of unit. The overall system noise figure is reduced by the
elimination of the circulator, it is much more stable, it can be electronically
tuned, its bandwidth is greater, and its saturation characteristic is improved.
In this method of operation (Fig. 6), the signal enters at one end of the
structure, travels along a transmission line past a slab of ruby, picking up gain
as it goes. The amplified signal leaves at the other end. In a cavity maser the
fields are built up by a resonant technique to enhance the interaction of the signal
with the crystal. In the TWM, the signal reacts very weakly with the crystal and
the gain per inch of structure is small. For a signal traveling at its normal speed,
the speed of light, the structure would have to be 50 to 70 feet long to attain
a gain of 20 db. This is not practical so the signal is slowed down by using a series
of metal rods forming a comb structure in which the signal is bounced back and forth
before it emerges at the output. The signal is then slowed down, and this increases
the reaction time between the signal and the ruby crystal. Gains of 25 db can be
obtained in lengths of 5 to 10 inches in sections of this type. This technique is
not new, it has been used very effectively in many devices. The traveling-wave tube
is an excellent example of a device using the same technique. The TWM is made oscillation-proof
by the inclusion of small ferrite isolators between each finger in the slow-wave
structure. These isolators look transparent to a signal traveling in the forward
direction but very "lossy" to a signal traveling in the reverse direction. This
eliminates any regenerative effects which are the primary cause of instability and
oscillations. The pump power is introduced into the crystal in the same resonant
manner as in the cavity maser.
Cryogenics
In order to operate a maser, the ruby crystal must be kept at a very low temperature,
usually at or below 4.2° absolute. The only substance that is liquid at such
low temperatures is helium. Liquid helium boils (that is, turns into a gas) at 4.2°
absolute. Because liquid helium boils at such a low temperature, elaborate systems
have had to be devised to contain it. In fact, the whole science of cryogenics has
developed around liquid helium, its production, storage, and effect on other materials
at very low temperatures.
Complete ruby maser developed for Signal Corps by Hughes. Assembly weighs 25
lbs. and is 30" high and 5" in diameter. Double vacuum assembly contains liquid
helium and nitrogen for supercooling a half-inch square ruby crystal to only a few
degrees above absolute zero. The ruby and a 12-ounce permanent magnet are inside
the structure at the very bottom of assembly.
Most masers employ a double Dewar system to establish the continuous cold necessary
for operation. A functional sketch of such a system is shown in Fig. 5 and
a number of stainless-steel double Dewars are shown in the photos. The double Dewar
is nothing more than one vacuum bottle inside another. The inner one is filled with
liquid helium and the outer is filled with liquid nitrogen to reduce dissipation
of the helium (liquid nitrogen boils at 77° absolute). The maser structure is immersed
in the liquid helium, supported on long narrow stainless-steel rods which reduce
boil off. With proper design, a single charge of liquid can last from 18 to 24 hours,
after this the Dewar system must be recharged. Cryogenic engineers are developing
a closed-cycle system which will allow continuous operation without recharging.
This type of system collects the helium gas as it boils off, re-liquefies it, and
returns it to the Dewar. When these closed systems become readily available, we
can expect to see greater use of masers. Currently, the open helium systems are
not very desirable - recharging is tricky and costly, storage of the liquids is
difficult, and the supply of liquid helium is very limited.
The operation of a TWM with a closed-loop refrigerating system forms a very practical
low-noise receiver. It can be remotely operated for extended periods of time without
maintenance.
Systems Applications
The systems applications of masers have been slow in coming, because many components
in present systems were not designed for use with an ultra-low-noise receiver. For
a maser to be useful, the noise contributions of the rest of the system should be
of the same order as that of the maser. It is for this reason that the Bell Telephone
Laboratories had to develop a special low-noise antenna for use with the maser for
project "Echo." This is the first system engineered to take maximum advantage of
the maser's characteristics.
Among the many systems that can make good use of the low-noise characteristics
of a maser are: preamplifiers for radio astronomy receivers, radar front ends, and
numerous systems that do not suffer from high background noise.
To take full advantage of a maser one would have to mount it directly at the
feed of an antenna. Giordmaine and Meyer of Columbia University operated a 9000-mc.
radio telescope with a maser preamplifier mounted on the feed of a 50-foot parabolic
reflector at the Naval Research Laboratory. The effective noise temperature of the
complete system was 85°K with the maser contributing about 4° maximum and the antenna
20°. The rest of the noise contributions were due to input losses and the second
stage. If a typical X-band mixer had been used instead of a maser, a noise temperature
of 20000K would not be uncommon. This shows an improvement on the order of 25 times
in the reduction in noise and hence increase in sensitivity with the use of a maser.
B. F. C. Cooper and J. V. Jelley operated a maser radio telescope at 1420 mc.
with the maser mounted at the feed of a 60-foot reflector located at the Harvard
College Agassiz Station. The overall noise characteristics of this maser system
were similar to those of the X-band maser radio telescope mentioned previously.
A unique feature of this system was a closed-loop feedback system to keep the maser
gain constant.
The use of a maser as a preamplifier for a radar receiver was first accomplished
by Hughes Aircraft Company. A 10-db improvement in the effective noise temperature
was obtained as compared to the normal receiver operation. The main problem with
a maser when used with a pulsed radar is that the maser saturates from pulse leakage.
To overcome this deficiency, a special low-loss ferrite switch had to be constructed.
This switch inserted about 30-db loss when the radar was in the transmitting state.
In the receiving state the switch loss was only about 0.25 db. The overall 10-db
improvement with the use of a maser almost doubled the range of the radar.
The maser has proven that it can detect and amplify signals better than any other
type of receiver. We can expect the maser to develop from a laboratory curiosity
to the workhorse of ultra-low-noise receiving systems. The major drawback to a maser
is the necessity of maintaining low temperature for operation. Hughes Aircraft has
operated a maser successfully at liquid nitrogen temperatures using the present
maser material (ruby).
In the future we can expect the development of new materials that will permit
operation at higher temperatures. For masers operating at 4.2°K, there is a recovery
time problem, which will only be corrected with the development of new maser materials.
There are a number of new, materials that look promising for 4.2°K and higher temperatures,
among them iron-doped sapphire and iron- or chromium-doped titanium dioxide (known
as rutile or titania). For frequencies above 10,000 mc., there is no other type
of amplifier that can approach, even closely, the extremely low noise and high sensitivity
of a maser. Masers have already been operated from 400 to 75,000 mc. Many laboratories
are trying to develop infrared and light masers.1 If successful, these
will be the first amplifiers for such types of electromagnetic radiation. These
are but a few of the things that can be expected. The future for this amazing receiver
is certainly beyond the skies.
See "The Laser - a Light Amplifier" in our September issue.
Posted April 16, 2020 (updated from original post on 12/31/2013)
|