March 1958 Radio-Electronics
[Table
of Contents]
Wax nostalgic about and learn from the history of early electronics.
See articles from Radio-Electronics,
published 1930-1988. All copyrights hereby acknowledged.
|
This second in a series of International
Geophysical Year (IGY) articles that appeared in Radio-Electronics magazine
in 1958.
The author covers basics of satellite configuration, launching, and tracking based
on knowledge of the era. Keep in mind, though, that the U.S. had not actually launched
its first satellite at the time. In fact, the two satellite models shown possess
antennas suggesting active radio circuits within, but
Echo, our
first passive earth-orbiting satellite, was just a metallized plastic sphere that
reflected radio signals back to Earth. The Russian
Sputnik, by
comparison, did have electronic circuitry onboard for transmitting but not receiving
a signal. SCORE, launched in December of 1958, was America's first transponder
satellite.
Electronics and the IGY
- Part II
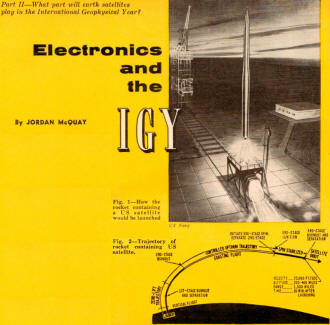
Fig. 1 - How the rocket containing a US Satellite would
be launched (upper figure, above). (U.S. Navy Photo)
Fig. 2 - Trajectory of rocket containing US satellite (lower
figure, above).

Fig. 3 - Amateur type setup for tracking earth satellites.
Part II: - What part will earth satellites play in the International Geophysical
Year?
By Jordan McQuay
In the search for scientific data during the IGY no single activity has stirred
the imagination and interest of the world more than the earth satellites - the rocket-borne
metal spheres sent into outer space to circle the earth, whirling free through an
orbit in upper atmosphere. Long considered a theoretical possibility, it was not
until recent development of rockets and missiles of tremendous size that this dream
has become a reality.
In October, 1957, the first satellites were launched by Russia. A series of satellites
will be launched by the United States during the late winter and spring of 1958.
Weighing about 185 pounds and about 2 feet in diameter, the Russian type of satellite
is launched into space by multiple-stage rockets of tremendous size and thrust.
Once it overcomes the gravitational pull of the earth at an altitude of several
hundred miles, the satellite circles the globe in an orbit 200-400 miles above the
earth at about 18,000 miles an hour. Altitude gradually drops over a period of several
weeks or months, and the satellite eventually disintegrates due to air friction.
Although technical details of the Russian satellite have not been revealed, it
contains a radio transmitter which broadcasts a coded signal (on 20 and 40 mc).
Since it is powered by some sort of miniature storage battery, failure of the power
supply after 4 or 5 weeks means the satellite moves silently through its orbit until
it disintegrates. General theory behind the Russian satellite, however, is much
the same as that of the several types of satellites soon to be launched by the United
States.
Satellite Principles
High-altitude rockets, described last month, provided the first direct experimental
observations of the upper atmosphere. These included data on air pressure, density,
temperature, composition, wind fields, cosmic rays and other solar activities. A
major limitation of these rockets is the brief period of time during which the measurements
could be taken - usually for only 6 or 7 minutes. Rocket coverage is also restricted
to a small part of the earth's atmosphere.
An artificial satellite, propelled into space and whirling in an orbit far above
the earth, will provide weeks, months, even years of continuous, reliable data for
scientific study. Also, the satellite traverses a vast amount of interplanetary
space during each revolution around the earth and thus collects a great amount of
geophysical information.
Once in its orbit, the satellite's velocity is such that its centrifugal force
balances the earth's gravitational pull. Without additional propelling power, the
satellite continues to circle the earth, making a complete revolution about once
every 80 or 90 minutes.
The principal problem is launching the satellite and propelling it upward into
its orbit. This is done by transporting the satellite in the nose of a multistage
rocket which has sufficient power to carry the satellite into the upper atmosphere.
The United States uses a three-stage rocket (Fig. 1). It is 72 feet long
and is launched vertically. Finless, it uses internal electronic controls for guidance.
The trajectory of the rocket is shown in Fig. 2.
When fired, the first stage of the rocket thrusts the entire assembly upward
almost vertically. It then tilts slightly until, at burnout, the rocket is inclined
at about 35°. Then, its fuel exhausted, the first stage detaches itself from
the rocket assembly. The second stage then drives the rocket to an altitude of about
140 miles, propelling it at a rapidly increasing speed to about 2,000 miles an hour,
and - through electronic controls - diminishes the angle off inclination to only
a few degrees. As the rocket levels off and coasts for some distance, the second
stage detaches itself and ignites the third and final stage of the rocket. The third
stage carries the satellite to its ultimate altitude of several hundred miles and
to its top speed of about 20,000 miles an hour. The satellite separates from the
third stage and, established in its orbit, continues under its own momentum - about
1,500 miles from the launching site and about 10 minutes after launching. Because
of its extreme speed at time of separation, the third stage may continue to orbit
somewhere in space behind the satellite. After some time, however, the third stage
will drop in altitude until it disintegrates in more dense atmosphere.
Although a satellite may continue to circle the earth for protracted periods
of time - several weeks, months or longer - ultimately atmospheric drag will bring
its orbit closer and closer to the earth. When it enters the denser atmosphere of
lower altitudes, the satellite (due to air friction) will burn out far above the
earth's surface. Both it and burned-out stages of the rocket will drift to earth
as indistinguishable dust and ashes.
While in flight, the tiny satellite broadcasts a periodic signal giving the specific
data it measures - such as air density, pressure, temperature .or solar activities.
The radio transmitter in each US satellite weighs about 13 ounces and has a 10-mw
output at a fixed frequency of 108 mc. It is crystal-controlled and completely transistorized.
Some types of satellites may have transmitters powered by seven 1.2-volt miniature
batteries. Others will be powered by solar batteries, which give the transmitter
a continuous life until the satellite eventually disintegrates.
The type of data transmitted by a satellite depends upon the instruments contained
within its spherical metal shell. Measurements are fed to electronic telemetering
equipment, which translates them into coded signals. Then the radio transmitter
broadcasts these signals to ground tracking and observing stations.
Tracking the Satellites
Once established in its orbit, a satellite must be tracked - both optically and
electronically - to provide position and path information to correlate with other
readings and measurements. With previous knowledge of the probable path of a satellite
gained through electronics, observers at ground stations can use photo-theodolites
for optical tracking. This method of tracking, however, depends upon fair visibility
for good accuracy.
A more reliable method of tracking is the Minitrack system, which utilizes radio
receiving equipment. The radio transmitter within the satellite produces a periodic
signal at 108 mc, which is radiated by small antennas outside the metal sphere.
On the ground, the signal can be detected by highly sensitive receiving equipment
whenever the satellite passes in the general vicinity of a receiving station.
Orbits of all US satellites are expected to have a latitude range of about 35°
above and below the equator. Within this broad belt, Minitrack stations have been
erected by many governments at strategic points around the world. Although development
and launching of the US satellite is primarily a contribution of this country to
the IGY, all countries are participating in observing and measuring data obtained
by each of the US satellites.

Fig. 4 - A miniature U.S. satellite. (U.S. Navy photo)

Fig. 5 - A conventional U.S. satellite in space.
Each ground station of the Minitrack system is equipped with several sets of
two specially designed and highly balanced receiving antennas, a frequency converter,
a high-gain amplifier and a visual recording device. When tuned to the 108-mc frequency
and with the satellite within receiving range, there will be an indication on the
output recording device - the amount depending on the proximity of the satellite
to the station. The satellite can be located in its orbit by comparing the signal
from one antenna with the signal from the second antenna of each set. This is equivalent
to comparing the path length of the signal from the satellite transmitter to one
receiving antenna with the path length of the signal to the second antenna of each
set of matched antennas. Similar measurements with other sets of matched antennas
at the receiving station will fix the satellite even more accurately.
A simplified version of the Minitrack system can be used by radio amateurs residing
in the region to be covered by each US satellite. As shown in Fig. 3, as few
as two balanced antennas are connected via a frequency converter, to the input of
a conventional communications receiver. An S-meter or other visual indicating device
is used at the receiver's output. As the satellite passes over the vicinity of the
station, the receiver output varies from a minimum to a peak. The maximum reading
determines the general position of the satellite. It recurs about every 90 minutes.
The equipment at each Minitrack station is considerably more complex and provides
a high degree of precision measurement. In addition, the satellite signal is continuously
recorded at each Minitrack station. In event of failure of the satellite's radio
transmitter, ground-based radar equipment tracks the satellite.
All tracking information is transmitted to key or central stations. There, using
instantly available data from all reporting sources, electronic computers calculate
orbital information and predict the exact path of any satellite for each successive
revolution. This prediction includes the time and place of meridian passage, the
zenith angle and the angular velocity of a satellite in its orbit. With each successive
revolution, these data are reevaluated and recomputed, and new predictions are made
by electronic data processing and computing equipment.
Data from the Satellites
As each satellite travels through space, specialized types of scientific data
are also obtained and measured by instruments within the sphere and then transmitted
to ground stations for collation and record.
These specialized data may relate to the sun's ultraviolet rays, meteor particles,
air density, cosmic rays, the ionosphere or any of many other fields of scientific
endeavor during the IGY. The type of data obtained and telemetered to earth by each
satellite depends entirely upon the type of instruments within the satellite. The
instrumentation is usually different for each of the US satellites, depending upon
its mission. By nature of their movement in space, however, all satellites provide
important scientific data concerning air density, the shape of the earth, the ionosphere
and other scientific fields.
Since the orbit of a satellite is influenced by local non uniformities in the
gravitational field, observations of the orbit at ground tracking stations make
possible calculations of mass distribution of the earth. This, in turn, yields information
about the composition of the earth's crust. Similar information, after electronic
analysis, also provides data about the oblateness or flatness of the earth near
the poles. Orbit observations also make possible precise determinations of latitude
and longitude, particularly for isolated islands, many of which in the Pacific have
never been located and mapped accurately.
Since radio signals from a satellite are affected as they pass through the ionosphere,
this phenomenon permits measurement of refraction as well a other characteristics
of the ionosphere. Such measurements are important to the study and prediction of
radio-wave propagation.
All types of data collected and recorded during the IGY - by Minitrack, optical
and other tracking stations as well as scientific observing stations - are transmitted
to key or central stations. There the various data are fed to electronic data processing
equipment for immediate or future reference.
From this mass of accumulated and correlated information, detailed an accurate
scientific data can be compiled electronically and almost instantly months, even
years, after a satellite has completed its flight through interplanetary space.
During this winter and spring, the United States will launch four miniature satellites.
These are trial flights primarily to test the Minitrack and rocket-launching systems.
Each of the test satellites is about 6 inches in diameter - the size of a grapefruit.
Each has six protruding antennas and contains a tiny radio transmitter powered by
solar batteries to convert energy from the sun into electricity. (See Fig. 4.)
Each will be launched by a conventional high-power three-stage rocket.
Larger satellites, to be launched during this summer, will be equipped to obtain
specialized scientific data. These are about 20 inches in diameter and weigh 21
pounds. Each is equipped with a transmitter and has four protruding antennas to
radiate data to the ground. (See Fig. 5.)
The first of the large satellites will carry instruments to study the sun's ultraviolet
rays and obtain environmental data. Succeeding satellites will record erosion of
meteor particles in space, measure air density and composition, the earth's magnetic
field, cosmic rays, and obtain other scientific data.
Other Studies
There are numerous other studies of scientific significance during the IGY. Simultaneous
studies in oceanography and glaciology are exploring the heat and water interrelationships
that also affect the earth's weather and climate. A study of seismology leads to
new knowledge of the earth's core and crust. Gravity measurements and related studies
are also part of the activities of all participating countries. But electronics
assists to only a very small degree in these international ventures.
Electronics, however, is widely utilized in most of these studies for recording,
filing and storing the wealth of data obtained.
At key control centers throughout the world, the latest types of electronic data-processing
equipment handle, record and store the vast amount of data collected continuously
during the IGY. Electronic computers are utilized for fast computation and analysis.
Data is recorded on punched cards or on metallic tape, then filed in electronic
storage memories for future reference. This makes the handling of billions of items
of measurement and observation largely automatic - through electronic processing.
The IGY is destined to yield unprecedented knowledge about the mysteries of the
earth and the atmosphere and their relationship to the sun. In geophysics, the universe
itself performs the experiments in which mankind is interested. The events that
determine our physical environment are therefore worldwide in nature, and only through
the cooperative efforts of all countries can their secrets be discovered.
Thus, through the joint effort of many nations, the International Geophysical
Year is not only an expression of the scientific interests of various countries,
but the scientific community of the world as a whole.
Posted November 24, 2021 (updated from original post on 2/27/2014)
|