June 1968 Radio-Electronics
[Table of Contents]
Wax nostalgic about and learn from the history of early electronics.
See articles from Radio-Electronics,
published 1930-1988. All copyrights hereby acknowledged.
|
Learn-at-home, self-taught curriculums were a big thing in the post-war
years of the 20th century. It was seen as a way to earn while you learn, where a
person worked a "day job" while studying to be a brain surgeon, nuclear
physicist, electrician, auto mechanic, or HVAC technician. It was more
convenient and less costly than driving to a campus for regular classes. Many
such courses were advertised in technical magazines like
Popular Mechanics,
Popular Science, and even
Radio-Electronics. This 1968 article entitled "Solid State Secrets" was
structured like one of those self-taught courses. Having paid for and completed
a couple of those courses, I can attest to their value, but ultimately I cannot
claim they contributed directly to any job offers. My USAF electronics training
got me into the civilian electronics world as a technician, and then earning a
BSEE degree advanced me into engineering. Note that the author elected to
feature
germanium as the semiconductor substrate rather than silicon, even though by
1968 the purification process for silicon had advanced to where it was
overtaking germanium in the industry.
Solid State Secrets
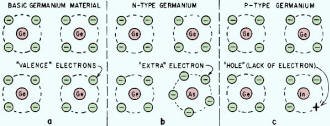
Fig. 1 - Atomic structure of semiconductor materials.
a - Pure germanium atoms have four electrons. b - N-type germanium
forms when arsenic doping adds electron. c - Indium causes electron hole.
A programmed course in semiconductor fundamentals
By Glenn M. Rawlings
Secrets of solid-state devices such as transistors, diodes, silicon controlled
rectifiers can be learned without too much difficulty. The programmed text that
follows is a modern self-teaching method designed to improve comprehension and retention
of the subject matter.
As you read through. each block section, and follow the directions given. you
will be led to a better understanding of solid-state principles.
Knowledge breeds knowledge ... if you can cope with this material chances are
that you will be stimulated toward additional investigation into the subject. Go
to Block 1 and follow the instructions.
To understand what a solid-state device is we must first analyze its general
construction. The term semiconductor is used with solid-state devices, Why? Because
the elements of which they are made have electrical characteristics somewhere between
an insulator and a conductor. Remember that a conductor is a material with many
"free" electrons, whereas an insulator has relatively fewer of these free electrons.
The two most common semiconductor materials are silicon and germanium. They are
similar in structure and the descriptions to follow are applicable to either in
most instances. Only the germanium material will be used for explanation.
In Fig. 1-a the atomic structure of germanium is seen in a simplified way. Orbiting
around the center core or nucleus of the atom are four valence electrons. These
valence electrons are the important thing to remember. They are rather loosely bound
to the center core. As you'll see later, they are used for current carriers.

Fig. 2-a - Zener diodes effectively regulate voltage. b -
Tunnel diode conduction curve.
Two basic modifications are performed on germanium during the semiconductor manufacturing
process. First, impurity atoms (such as arsenic) are doped with the basic germanium
atoms. The impurity atoms in this case have, not four, but five valence electrons
in their orbit. As seen in Fig. 1-b, this causes the basic material to have a few
extra electrons distributed within its structure. You recall that electrons by definition
are negative charges? Then it should be easy to remember that this material is n-type.
In the second modification to the basic germanium material, impurity atoms (such
as indium) are doped in the same manner as before. The impurity atoms of indium
have only three valence electrons in their orbit. As seen in Fig. 1-c, the material
now has a number of empty "pockets" or "holes" distributed within its structure.
It has fewer electrons than the n-type material of Fig. 1-b; therefore by definition
it is a more positive material. We call it p-type material.
Question: Which of the following best describes an n-type germanium
material?
_ It has fewer electrons than normally found in the basic germanium material.
Go to Block 7.
_ It is an insulator. Go to Block 20.
_ It has more electrons than normally found in the basic germanium material.
Go to Block 8.
Your answer is wrong! Return to Block 3 and restudy. Select
another answer.
Would you believe ... you're right! The holes in a sense, are "recombined" with
electrons from the n-type material.
A simple Zener diode voltage regulator consists of a voltage source, a current-limiting
resistor and a Zener diode selected for the desired output voltage. Refer to the
schematic of Fig. 2-a. The arrow of the diode always points to the positive voltage
source in a circuit such as this. If the output load decreases, the Zener current
will increase to maintain a constant output voltage. This is a very simple and effective
regulation circuit.
Zener diodes are available with breakdown (operating) voltages anywhere from
2 to several hundred volts. An important parameter of Zener regulators is wattage
rating. The wattage is determined by the product of the Zener voltage and maximum
Zener current for any particular application.
What else can we do with these p- and n-type materials? Another item we should
briefly mention is the tunnel diode. Its construction is identical to an ordinary
diode's, with one exception: more impurity atoms are added to the basic material.
This causes the diode's forward conduction characteristic to appear as in Fig. 2-b.
Section A is a "negative-resistance" region. Notice that the current actually decreases
while the voltage is increasing. This characteristic of the tunnel diode is used
for many applications, especially in the amplification of high-frequency signals.

Fig. 3 - Load line (AB) is drawn on transistor collector
operating curves to find circuit operating characteristics, then dc operating point
is selected.
Question: If the Zener diode in Fig. 3-a is a 10-volt device
and the maximum current through it is 1 ampere, what is its wattage dissipation?
_ 1 watt Go to Block 19.
_ 3 watts Go to Block 2.
_ 10 watts Go to Block 11.
Your answer is ... Correct!
It could get pretty confusing if we tried to analyze an amplifier under its dynamic
conditions without some type of graph or chart to tell us what happens at any specific
time. We know the collector current, base current and collector voltage are all
related to each other. For instance, if base current is increased, collector current
increases and collector-to-emitter voltage decreases. This can be seen by referring
to the transistor "load-line" drawing of Fig. 3.
The load line, once established, will tell us the dynamic operating characteristics
of a particular transistor type. The transistor manufacturer usually supplies the
basic graph and the user then plots the load line to suit his requirements. Much
knowledge of transistor action can be obtained by learning the fundamentals of this
graph. The steps used to draw the load line of Fig. 3 are:
1. Determine collector voltage when collector current is zero (With no collector
current flowing, there is no voltage drop across the 1200-ohm resistor. Hence the
full -30 volts appears at the collector. This is shown as point A)
2. Determine collector current when collector voltage is zero. (If the collector
is at zero volts the transistor would have to be turned full on. Thus for practical
purposes, the 1200-ohm resistor is the only limit to collector current.
Since I = E/RE then I = 30 volts/1200 ohms
= 25 mA
3. Draw the load line between points A and B.
4. Determine a dc operating point on this line. (A linear area is usually chosen
where the base-current change is equal on each side . of the operating point.)
Refer to Fig. 3. You will see that when the base-current change is 20 μA, the
collector current will change a total of 12 mA. Likewise, the change in collector
voltage will be 15 volts.
This is a good time to mention two terms encountered in transistor theory. The
first is beta (β), which is simply the ratio between the base-to-emitter current
and the collector current. In our example, it would be 12mA 12 mA/20 μA = 600. This
example would represent a very high-gain transistor circuit.
The second term encountered is alpha. This is the ratio between the collector
and emitter currents. Since these currents are almost identical (the emitter contains
the small additional base current) this ratio is generally close to unity.

Fig. 4-a - Field-effect transistors (FETs) have advantage
of high input impedance. Negative bias on gate (G) is used to regulate electron
flow (arrows). b - Arrow direction on gate indicates this is an n-channel
FET. If arrow is reversed, it is p-channel FET with p-type material in its body.
Question:
If the base current of a transistor is increased, the collector current will
decrease.
_ True. Go to Block 9.
_ False. Go to Block 15.
Your answer is right ... for a transistor (ordinary two-junction type), but not
for a unijunction transistor. A unijunction has one emitter and two base leads.
Go to Block 6.
You are correct.
One problem when using ordinary transistors in certain circuits is the loading
effect they present. For instance, it is difficult to match the high impedance of
a crystal transducer to the considerably lower input impedance of a conventional
transistor base circuit. This problem is eliminated by the field-effect transistor
(FET). Just as its name implies, it uses a field as shown in Fig. 4-a to control
current :flow through its main section. As shown, the drain is made positive with
respect to the source, so electrons flow from source to drain. The gate is biased
negative - and the more negative it becomes, the more the field blocks current flow
to the drain.
Operation is very similar to a vacuum-tube grid; each has a very high input impedance.
If the FET body is made of n-type material, the device is called an n-channel
FET. If the body is made of p-type material, it is called a p-channel FET. FET elements
are called source, gate and drain. They perform much the same functions as their
names imply.
Fig. 4-b shows a typical n-channel FET amplifier circuit. If the arrow on the
gate lead were pointing in the other direction, the device would be a p-channel
type. Remember the great similarity between the field-effect transistor and its
grandfather, the vacuum tube.

Fig. 5-a - With positive battery terminal connected to anode,
electrons flow to p material, holes move to n material. b - No current flows
when polarities are reversed (c); if voltage is increased to breakdown point, current
jumps sharply.
Question: An important characteristic of the field effect transistor
is ...
_ Its ability to open a gate and drain a source.
Go to Block 14.
_ Its high output impedance. Go to Block 16.
_ Its high input impedance. Go to Block 21.
Sorry about that ... Go back to Block 1 and refer to Fig. 1-b.
Select another answer.
Your answer is right!
And now that you know what n-type and p-type semiconductor materials are, you
may well say, "So what?" Let's take a block of n-type and a block of p-type germanium
and place them together, as shown in Fig. 5-a. The positive terminal of the battery
is connected to the p-type material, the negative terminal to the n-type. Now the
fun begins. The junction is said to be forward-biased and the "extra" electrons
in the n-material will move in the direction of the junction. Since they are loosely
bound in the valence bond, they may leave each nucleus rather easily. When a free
electron reaches the junction, it will exchange places with a hole. (That is, it
will fill a hole in the p-type material and leave a hole in the n-type material
from whence it came.)
This always sounds a little confusing at first. Just remember that the electrons
flow from the n- to the p-type material, and the hole flow is the opposite. Some
of you younger readers may call this exchange of positions a "happening." Actually
it's just what takes place in an ordinary solid-state diode.
Fig. 5-b shows the characteristic curve for a typical diode. The amount of forward
current that flows is directly dependent upon the forward voltage applied across
the diode terminals.
If we reverse the battery connections to the n- and p-type materials - as in
Fig. 5-c, a very interesting thing takes place. The free electrons and holes are
actually pulled away from the junction. Current flow ceases, except for a very small
leakage current. Obviously the diode conducts in only one direction.
So far, we have been assuming the reverse voltage applied to the diode is insufficient
to cause a breakdown of the pn junction. If we continue increasing this voltage,
however, a breakdown will take place - as shown in Fig. 5-b. A heavy current flows
and, due to the sharpness of the breakdown, a very small voltage change takes place.
This characteristic is used in Zener or breakdown diode applications. Since silicon
gives the sharpest breakdown curve, this material is used for most Zener diodes.

Fig. 6-a - Internal make-up and circuit connections for
three-layer npn transistor. Junction A is reverse biased, junction B is forward
biased. b - Schematic notation for npn transistor circuit.
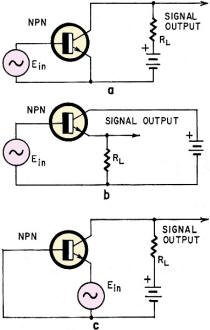
Fig. 7 - Three commonly used amplifier circuits. a -
Common emitter has high voltage, current and power gain. b - Common collector: no
voltage gain, high current and low power gain. c - Common base: high voltage gain,
low current gain, and medium power gain.
Question: When a pn junction is forward-biased, the holes do
which of the following:
_ Flow away from the junction. Go to Block 18.
_ Flow into the junction where they are subsequently occupied by an electron
from the n-type material. Go to Block 3.
_ Remain stationary. Go to Block 12.
Your answer is not correct. Return to Block 4, study Fig. 3
and then go directly to Block 15.
Wrong! Go to Block 11 and select another answer.
Your answer is correct! If the Zener has a 10-volt drop across it and 1 ampere
of current, the dissipation is 10 watts (P = IE). For this example, at least a 15-watt
Zener would be used.
By now you are probably wondering what happened to the good old transistor encountered
in everything these days. So far we have been discussing semiconductor devices that
are constructed of one slice of n-type material and one slice of p-type material.
These can all be classified as two-layer diodes. Suppose we sandwich a thin slice
of the p-type material between two pieces of n-type, as shown in Fig. 6-a. With
the battery voltage applied as shown, junction A has a reverse bias applied to it.
Junction B is forward-biased. The current that will flow through resistor RL
depends on the amount of bias at these two junctions. If VBE is increased
(as would be the case with an input signal) current through RL will increase.
A pnp transistor is constructed in the same manner as the npn, except that a
thin slice of n-type material is sandwiched between two of p-types, The operation
of both transistor types is identical. In fact, an npn may be directly interchanged
with a pnp (of similar characteristics) if the supply voltage polarity is reversed.
(In practice this is seldom done, as polarized capacitors might be damaged.)
An important thing to remember about transistors is that the base-to-collector
junction is reverse-biased, while the base-to-emitter is forward-biased. For any
normal transistor configuration this must be true. Notice that Fig. 6-b is the schematic
version of Fig. 6-a and represents the bias conditions just mentioned.
Fig. 7 shows the three most common amplifierss used in transistor work. Note
the characteristics associated with each. A great deal of practical knowledge can
be gained by becoming familiar with these three configurations.
Question: In a typical transistor circuit, which of the following
statements would be correct?
_ The base-to-emitter junction is reverse-biased, while the base-to-collector
is forward-biased. Go to Block 17.
_ The base-to-emitter is forward-biased, while the base-to-collector is reverse-biased.
Go to Block 4.
_ Both the base-to-emitter and base-to-collector junctions are forward-biased.
Go to Block 10.
Would you believe ... Wrong! For our purposes, it is best to consider the holes
as actually moving. Return to Block 8 and select another answer.
Sorry about that ... your answer is not correct. Go back and review Block
15 and then select another answer.
In a sense this may be true, but it is not the correct answer here. Better return
to Block 6 and select another answer.
You are absolutely right!
Another type of semiconductor device is the unijunction transistor (UJT). This
device has no collector element in the normal sense. It consists instead of two
base leads and one emitter. Its construction is shown in Fig. 8-a. The body consists
of a piece of n-type material. The resistance of this silicon is relatively high
between the base 1 and base 2 connections; normally very little current will flow
between these elements.
But suppose we apply a voltage between the emitter and base 1 junction - a voltage
high enough to forward-bias the junction. Then the resistance between the emitter
and base 1 becomes very small. When this happens, the resistance of the body (from
base 1 to base 2) is suddenly lowered and current flow increases. This effect is
put to good use in timing and pulse-generation circuits. As an example, some recent
video pattern generators use a UJT for timing functions.

Fig. 8-a - Unijunction transistor has two base leads and
one emitter. Normally high resistance between B1 and B2 can be quickly lowered by
emitter voltage, permit-ting current to flow. b - Timing circuit utilizes this
resistance-breakdown effect.

Fig. 9-a - A four-layer diode, or pnpn switch. At certain
level of applied voltage across terminals junction 2 breaks down, resulting in very
low resistance between anode and cathode. Current pulse that results is available
as an output. b - In thyristor or silicon controlled rectifier, this breakdown
is controlled by bias on the gate.
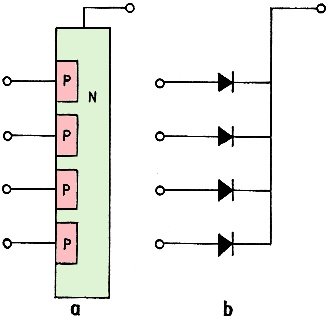
Fig. 10-a - Basic integrated circuit (IC) consists of n-type-material
with 4 p-type junctions. b - Schematic of (a) shows four diodes with cathodes
common.
Fig. 8-b shows the unijunction transistor symbol and a typical timing circuit.
As capacitor C1 charges through resistor R1, the voltage eventually becomes great
enough to forward-bias the emitter-to-base 1 junction. This causes the capacitor
to discharge suddenly through base 1 and resistor R2. When the capacitor discharges,
the cycle starts all over again. The values of R1 and C1 determine the time between
output pulses.
After seeing the various configurations and arrangements of the two basic n-
and p-type materials and their applications, can there be more? Yes! You remember
that a pnp transistor is made of a thin slice of n-type material sandwiched between
two p-types, Suppose we add another n-type to this arrangement, as shown in Fig.
9-a. This is called a four-layer diode, or pnpn switch.
By taking advantage of the biased junctions and leakage currents across these
junctions, several valuable devices have been developed. The first is exactly as
shown in Fig. 9-a. When the applied voltage across this four-layer device reaches
a certain level, junction 2 will actually break down, and a very low resistance
will exist across the terminals. The point at which this occurs is relatively sharp
and a current pulse is available as an output.
The characteristics of a four-layer diode are similar to a thyratron tube; each
has a distinct firing (or breakdown) point. Once the device is turned on, it will
remain on until the anode voltage is removed or reduced to a very low level. The
breakdown may be controlled by attaching another lead as shown in Fig. 9-b. This
device is known as a thyristor or silicon controlled rectifier (SCR). It has found
its way into a multitude of uses such as electronic switching, motor speed control,
light dimmers, etc. Like the thyratron's control grid, the SCR's gate loses control
once conduction takes place.
Question: The unijunction transistor consists of which of the
following?
_ Two emitters and one base lead. Go to Block 13.
_ One emitter and two base leads. Go to Block 6.
_ One emitter, one collector and one base lead. Go to Block 5.
Your answer is wrong! Return to Block 6, restudy and try again.
Would you believe ... wrong. Return to Block 11 and try again.
Your answer is not correct. A reverse bias would cause the positive holes to
flow away from the junction. Return to Block 8 and try again.
Your answer is incorrect. As described in Block 3, the wattage
is the product of the Zener voltage and current (10 watts). Go directly to
Block 11.
Your answer is wrong. Return to Block 1 and select another answer.
You have chosen the correct answer once again. Since we began at Block
1, we have touched upon ordinary diodes, four-layer diodes, silicon controlled
rectifiers, ordinary transistors, unijunction and field-effect transistors, and
Zener and tunnel diodes. All these do a better job and take up less space than we
could have imagined just a few years ago. But even so, the latest trend is even
more amazing. Someone apparently opened the case of a transistor and discovered
a great deal of wasted space there. In the integrated circuit many components are
now put inside a case the size of a single transistor. These components are all
made of semiconductive materials like those we have been discussing. Transistors,
capacitors, diodes and resistors are constructed on a small chip that would fit
under your thumbnail.
Figure 10-a shows a very simple integrated circuit of four diodes with common
cathode connections. Fig. 10-b is the same circuit shown schematically.
Use your imagination and you will see that many entire circuits could (and in
fact are) constructed in a very small area. This technique is the future of electronics.
And after this, you may wonder what could possibly come next. Would you believe
... L.S.D.?*' R-E
*Liquid-state diodes
|