Module 18 - Radar PrinciplesPages
i,
1-1, 1-11,
1-21,
1-31,
1-41,
2-1,
2-11,
2-21,
2-31,
2-41,
3-1,
3-11,
3-21,
4-1,
4-11,
4-21,
AI-1,
AII-1,
Index-1 to 3

Figure 3-10. - Deflection coil currents.
CRT Screen Persistence A PPI requires a CRT in which the screen is coated with a
long-persistence phosphor. This is necessary because each target reflects energy for only a short period of time
during each rotation of the antenna. Therefore, the target indication on the face of the CRT must be able to
continue to glow during the portion of antenna rotation when the target is not reflecting energy. Q4.
What coordinates are presented on a PPI scope? Q5. What type of deflection is preferred for a CRT
electron beam? Q6. Which of the two types of deflection coils (fixed or rotating) is used most often?
RANGING Circuits The accuracy of target-range data provided by a radar varies with
the use of the radar. For example, a weapons systems radar operating in a search mode is required to be accurate
within a small percentage of its maximum range. However, an intercept radar, operating in a tracking mode, must
supply range data that is even more accurate; it must be within a few yards of the actual range. In some
applications of radar, the indicator sweep is calibrated by a transparent overlay with an engraved range scale.
This overlay enables the operator to estimate the range of targets. In other applications, electronic range marks
are supplied to the indicator. They usually appear as vertical pulses
3-11
on A-scopes and as concentric circles on PPI scopes. The distance between range marks is generally
determined by the type of equipment and its mode of operation. In a weapons systems radar that requires
extremely accurate target-range data, a movable range marker may be used. The range marker is obtained from a
range-marker generator and may be a movable range gate or range step. When a PPI scope is used, a range circle of
adjustable diameter may be used to measure range accurately. In some cases, movement of the range marker is
accomplished by adjusting a calibrated control from which range readings are obtained. The following
discussion describes the operation of three types of range-marker generators: the RANGE-GATE GENERATOR, the
RANGE-MARKER GENERATOR, and the RANGE-STEP GENERATOR. The range-gate generator, used in conjunction with a
blocking oscillator, generates a movable range gate. The range-marker generator and the range-step generator, used
in conjunction with an astable multivibrator, generate fixed range marks and a movable range step, respectively.
Range-Gate Generator Figure 3-11 shows a simplified block diagram of a typical range-gate generator.
The pulse-repetition frequency is controlled by a master oscillator, or multivibrator, in which the output is
coupled to a trigger thyratron (both in the synchronizer). The output of the trigger thyratron is used to trigger
the radar modulator and the scope sweep circuits, thus starting the transmitter pulse and the range sweep at the
same instant, referred to as time T0. 
Figure 3-11. - Range-gate generator.
The PHANTASTRON in the sweep circuits is a variable timing circuit that supplies a sweep sawtooth to the sweep
amplifier. The width of the gate and sawtooth is dependent upon the range selected by the radar operator. The range-gate circuit receives its input pulse from the trigger thyratron and generates a delayed range-gate
pulse. The delay of this pulse from time T0 is dependent on either the range of the target when 3-12
the radar is tracking, or the manual positioning of the range-volts potentiometer when the radar is
not tracking (in the search mode). The range-gate triggers the range-strobe multivibrator, from which the output
is amplified and sent to the blocking oscillator (which sharpens the pulses), as shown in figure 3-11. This range
gate is used to select the target to be tracked. When in the track mode, the range gate brightens the trace or
brackets the blip (depending on the system) to indicate what target is being tracked. Range-gate generators are used most often in weapons-control track radar A-scope presentations, but they can also be used with PPI
presentations. When used with a PPI presentation, the range gate must be movable in both range and bearing. The range-gate generator can easily be modified to produce a range strobe instead of a range gate. a range strobe
is simply a single brightened spot that is movable both in range and bearing. In operation, the range strobe or
range gate control also controls a dial or digital readout to provide a range readout to the operator. Range-Marker Generator Several types of range-marker generators are in common use. Figure
3-12 shows a simplified version of a circuit that produces both range markers and the basic system timing
triggers. The master oscillator in this case is a blocking oscillator that operates at a frequency of 80.86
kilohertz. By dividing 80.86 kilohertz into 1 (t = 1/frequency), we find the time required for one cycle of
operation is 12.36 microseconds. Thus the blocking oscillator produces pulses 1 radar mile apart. These are fed to
the 5:1 divider circuit. Five of the 1-mile marks are required to produce an output from the divider circuit.
These five-mile marks are sent to the indicator for display and to the 10:1 divider circuit. In the latter case,
ten of the five-mile marks are required to produce an output from the 10:1 divider. Thus the output triggers are
50 miles apart. These basic timing triggers are for a radar with a range of fifty miles. The period between
triggers could be extended through the use of additional dividers for use with longer range systems. 
Figure 3-12. - Range-marker generator. Another version of a range-mark generator is shown in figure 3-13. This circuit provides range marks at
1,000-, 2,000-, or 3,000-yard intervals. Generation of the marks begins with the ringing oscillator, which is
started by a delayed master trigger from the synchronizer. a ringing oscillator produces a sinusoidal output of a
fixed duration and frequency when triggered. The output is synchronized to the input trigger. In this circuit, the
trigger causes the oscillator to produce a 162- kilohertz signal that lasts for 4 1/2 cycles. The emitter follower
isolates the ringing oscillator from the countdown multivibrator and clips the oscillator output signals. This
action allows only the positive half of each sine wave to reach the multivibrator. The positive triggers from the
ringing oscillator are at 1,000- yard intervals. This input signal results in an output from the countdown
multivibrator of 1,000-, 2,000-, or 3,000-yard range marks, depending on the position of the RANGE MARK SELECT
Switch. 3-13

Figure 3-13. - Range-marker generator. Range-Step Generator The range step is often used to determine target range on an
A-scope presentation. The appearance of a range step on an A-scope is illustrated in figure 3-14. 
Figure 3-14. - Range-step presentation. View a of figure 3-15 is a block diagram of a simple range-step generator consisting of a sawtooth
generator, a negative clipper, a range potentiometer, and a limiting amplifier. The position of the range step
along the indicator's time base is controlled by the range potentiometer. When the range step coincides with the
leading edge of a target's echo pulse, the range can be read directly from a calibrated readout associated with
the potentiometer.
3-14

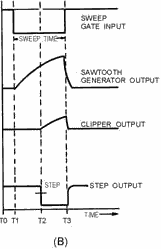
Figure 3-15. - Range-step generation. View B shows the time relationships of the voltage waveforms produced by the range-step generator.
During the sweep gate, the sawtooth generator produces a sawtooth voltage that is sent to the clipper. The point
at which the sawtooth is clipped is controlled by the range potentiometer. The clipped sawtooth is shaped in the
limiting amplifier to produce the output voltage waveform. The portion of the output waveform from T1 to T3 is
applied to the vertical-deflection plates of the indicator CRT to produce the display shown in figure 3-14.
Q7. What type of ranging circuit is most often used with a radar that requires extremely accurate range data?
Q8. The range sweep in a range-gate generator is started at the same time as what other pulse?
Q9. Range-marker generators produce pulses based on what radar constant? Q10. What radar scope uses a
range step for range measurement? 3-15
Radar Antennas In this section, we will briefly review the requirements of radar antennas. Antenna characteristics are
discussed in detail in NEETS, Module 10, Introduction to Wave-Generation, Transmission Lines, and Antenna and in
Module 11, Microwave Principles. a review of these modules would be helpful at this point to prepare you for the
following radar antenna discussion. Antennas fall into two general classes, OMNIDirectIONAL and
DirectIONAL. Omnidirectional antennas radiate energy in all directions simultaneously. They are seldom used with
modern radars, but are commonly used in radio equipment, in IFF (identification friend or foe) equipment, and in
countermeasures receivers for the detection of enemy radar signals. Directional antennas radiate RF energy in
patterns of LOBES or BEAMS that extend outward from the antenna in one direction for a given antenna position. The
radiation pattern also contains minor lobes, but these lobes are weak and normally have little effect on the main
radiation pattern. The main lobe may vary in angular width from one or two degrees in some radars to 15 to 20
degrees in other radars. The width depends on the system's purpose and the degree of accuracy required. Directional antennas have two important characteristics, DirectIVITY and Power Gain. The directivity of an antenna
refers to the degree of sharpness of its beam. If the beam is narrow in either the horizontal or vertical plane,
the antenna is said to have high directivity in that plane. Conversely, if the beam is broad in either plane, the
directivity of the antenna in that plane is low. Thus, if an antenna has a narrow horizontal beam and a wide
vertical beam, the horizontal directivity is high and the vertical directivity is low. When the
directivity of an antenna is increased, that is, when the beam is narrowed, less power is required to cover the
same range because the power is concentrated. Thus, the other characteristic of an antenna, power gain, is
introduced. This characteristic is directly related to directivity. Power gain of an antenna is the ratio
of its radiated power to that of a reference (basic) dipole. Both antennas must have been excited or fed in the
same manner and each must have radiated from the same position. a single point of measurement for the power-gain
ratio must lie within the radiation field of each antenna. An antenna with high directivity has a high power gain,
and vice versa. The power gain of a single dipole with no reflector is unity. An array of several dipoles in the
same position as the single dipole and fed from the same line would have a power gain of more than one; the exact
figure would depend on the directivity of the array. The measurement of the bearing of a target, as
detected by the radar, is usually given as an angular position. The angle may be measured either from true north
(true bearing), or with respect to the bow of a ship or nose of an aircraft containing the radar set (relative
bearing). The angle at which the echo signal returns is measured by using the directional characteristics of the
radar antenna system. Radar antennas consist of radiating elements, reflectors, and directors to produce a narrow,
unidirectional beam of RF energy. a pattern produced in this manner permits the beaming of maximum energy in a
desired direction. The transmitting pattern of an antenna system is also its receiving pattern. An antenna can
therefore be used to transmit energy, receive energy, or both. The simplest form of antenna for measuring azimuth
(bearing) is a rotating antenna that produces a single-lobe pattern. The remaining coordinate necessary to
locate a target in space may be expressed either as elevation angle or as altitude. If one is known, the other can
be calculated from basic trigonometric functions. a method of determining the angle of elevation or the altitude
is shown in figure 3-16. The slant range is obtained from the radar scope as the distance to the target. The angle
of elevation is the angle between the axis of the radar beam and the earth's surface. The altitude in feet is
equal to the slant range in feet multiplied by the sine of the angle of elevation. For example if the slant range
in figure 3-16 is 2,000 feet 3-16
and the angle of elevation is 45 degrees, the altitude is 1,414.2 feet (2,000 ´ .7071). In some radar
equipments that use antennas that may be moved in elevation, altitude determination is automatically computed.

Figure 3-16. - Radar determination of altitude. PARABOLIC ReflectorS A SPHERICAL WAVEFRONT spreads out as it travels and produces a
pattern that is neither too sharp nor too directive. On the other hand, a Plane wavefront does not spread out
because all of the wavefront moves forward in the same direction. For a sharply defined radar beam, the need
exists to change the spherical wavefront from the antenna into a plane wavefront. a parabolic reflector is one
means of accomplishing this. Radio waves behave similarly to light waves. Microwaves travel in straight
lines as do light rays. They may be focused and/or reflected just as light rays can. In figure 3-17, a
point-radiation source is placed at the focal point F. The field leaves this antenna with a spherical wavefront.
As each part of the wavefront reaches the reflecting surface, it is shifted 180 degrees in phase and sent outward
at angles that cause all parts of the field to travel in parallel paths. Because of the shape of a parabolic
surface, all paths from F to the reflector and back to line XY are the same length. Therefore, all parts of the
field arrive at line XY the same time after reflection.

FiFigure 3-17. - Parabolic reflector radiation.
3-17
If a dipole is used as the source of radiation, there will be radiation from the antenna into space
(dotted lines in figure 3-17) as well as toward the reflector. Energy that is not directed toward the paraboloid
has a wide-beam characteristic that would destroy the narrow pattern from the parabolic reflector. This occurrence
is prevented by the use of a hemispherical shield (not shown) that directs most radiation toward the parabolic
surface. By this means, direct radiation is eliminated, the beam is made sharper, and power is concentrated in the
beam. Without the shield, some of the radiated field would leave the radiator directly. Since it would not be
reflected, it would not become a part of the main beam and thus could serve no useful purpose. The same end can be
accomplished through the use of a PARASITIC array, which directs the radiated field back to the reflector, or
through the use of a feed horn pointed at the parraboloid. The radiation pattern of a parabola contains a
major lobe, which is directed along the axis of revolution, and several minor lobes, as shown in figure 3-18. Very
narrow beams are possible with this type of reflector. View a of figure 3-19 illustrates the parabolic reflector. 
Figure 3-18. - Parabolic radiation pattern. Truncated Paraboloid View B of figure 3-19 shows a horizontally truncated
paraboloid. Since the reflector is parabolic in the horizontal plane, the energy is focused into a narrow
horizontal beam. With the reflector truncated, or cut, so that it is shortened vertically, the beam spreads out
vertically instead of being focused. Since the beam is wide vertically, it will detect aircraft at different
altitudes without changing the tilt of the antenna. It also works well for surface search radars to overcome the
pitch and roll of the ship. 3-18

Figure 3-19. - Reflector shapes. The truncated paraboloid reflector may be used in height-finding systems if the reflector is rotated 90
degrees, as shown in view C. Because the reflector is now parabolic in the vertical plane, the energy is focused
into a narrow beam vertically. With the reflector truncated, or cut, so that it is shortened horizontally, the
beam spreads out horizontally instead of being focused. Such a fan-shaped beam is used to determine elevation very
accurately.
Orange-Peel Paraboloid A section of a complete circular paraboloid, often called an
ORANGE-PEEL Reflector because of its shape, is shown in view D of figure 3-19. Since the reflector is narrow in
the horizontal plane and wide in the vertical, it produces a beam that is wide in the horizontal plane and narrow
in the vertical. In shape, the beam resembles a huge beaver tail. This type of antenna system is generally used in
height- finding equipment. Cylindrical Paraboloid When a beam of radiated energy
noticeably wider in one cross-sectional dimension than in the other is desired, a cylindrical paraboloidal section
approximating a rectangle can be used. View E of figure 3-19 illustrates this antenna. a parabolic cross section
is in one dimension only; therefore, the reflector is directive in one plane only. The cylindrical paraboloid
reflector is either fed by a linear array of dipoles, a slit in the side of a waveguide, or by a thin waveguide
radiator. Rather than a single focal point, this type of reflector has a series of focal points forming a straight
line. Placing the radiator, or radiators, along this focal line produces a directed beam of energy. As the width
of the parabolic section is changed, different beam shapes are obtained. This type of antenna system is used in
search and in ground control approach (GCA) systems.
Q11. Which of the two general classes of antennas is most often used with radar? Q12. The
power gain of an antenna is directly related to what other antenna property? Q13. a parabolic reflector
changes a spherical wavefront to what type of wavefront? 3-19
CORNER Reflector The corner-reflector antenna consists of two flat conducting
sheets that meet at an angle to form a corner, as shown in view F of figure 3-19. This reflector is normally
driven by a half-wave radiator located on a line which bisects the angle formed by the sheet reflectors.
BROADSIDE ARRAY The desired beam widths are provided for some vhf radars by a broadside array, such
as the one shown in figure 3-20. The broadside array consists of two or more half-wave dipole elements and a flat
reflector. The elements are placed one-half wavelength apart and parallel to each other. Because they are excited
in phase, most of the radiation is perpendicular or broadside to the plane of the elements. The flat reflector is
located approximately one-eighth wavelength behind the dipole elements and makes possible the unidirectional
characteristics of the antenna system.
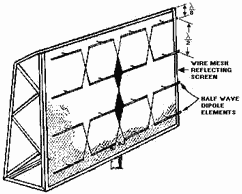
Figure 3-20. - Broadside array. HORN Radiators Horn radiators, like parabolic reflectors, may be used to obtain
directive radiation at microwave frequencies. Because they do not involve resonant elements, horns have the
advantage of being usable over a wide frequency band. The operation of a horn as an electromagnetic
directing device is analogous to that of acoustic horns. However, the throat of an acoustic horn usually has
dimensions much smaller than the sound wavelengths for which it is used, while the throat of the electromagnetic
horn has dimensions that are comparable to the wavelength being used. Horn radiators are readily adaptable
for use with waveguides because they serve both as an impedance-matching device and as a directional radiator.
Horn radiators may be fed by coaxial or other types of lines. Horns are constructed in a variety of shapes
as illustrated in figure 3-21. The shape of the horn, along with the dimensions of the length and mouth, largely
determines the field-pattern shape. The ratio of the horn length to mouth opening size determines the beam angle
and thus the directivity. In general, the larger the opening of the horn, the more directive is the resulting
field pattern. 3-20
- |
Matter, Energy,
and Direct Current |
- |
Alternating Current and Transformers |
- |
Circuit Protection, Control, and Measurement |
- |
Electrical Conductors, Wiring Techniques,
and Schematic Reading |
- |
Generators and Motors |
- |
Electronic Emission, Tubes, and Power Supplies |
- |
Solid-State Devices and Power Supplies |
- |
Amplifiers |
- |
Wave-Generation and Wave-Shaping Circuits |
- |
Wave Propagation, Transmission Lines, and
Antennas |
- |
Microwave Principles |
- |
Modulation Principles |
- |
Introduction to Number Systems and Logic Circuits |
- |
- Introduction to Microelectronics |
- |
Principles of Synchros, Servos, and Gyros |
- |
Introduction to Test Equipment |
- |
Radio-Frequency Communications Principles |
- |
Radar Principles |
- |
The Technician's Handbook, Master Glossary |
- |
Test Methods and Practices |
- |
Introduction to Digital Computers |
- |
Magnetic Recording |
- |
Introduction to Fiber Optics |
Note: Navy Electricity and Electronics Training
Series (NEETS) content is U.S. Navy property in the public domain. |
|