Module 7 - Introduction to Solid-State Devices and Power Supplies
Pages i ,
1-1,
1-11,
1-21,
1-31,
1-41,
2-1,
2-11,
2-21,
2-31,
2-41,
2-51,
3-1,
3-11,
3-21,
3-31,
3-41,
3-51,
4-1 to 4-10,
4-11,
4-21,
4-31,
4-41,
4-51, Index
These NEETS
training modules created by the U.S. Navy appear to have originally come out in
1990s and has been modernized a few times since then, so the information is
fairly up to date. You will still find a lot of descriptions of vacuum tubes,
but that is because even in 1998 the military still had a lot of legacy equipment
that needed to be maintained. This particular module provides the student with an
introduction to and history of semiconductor physics. The chapter runs a total of
62 pages so there's a lot of content.
Chapter 1 - SEMICONDUCTOR DIODES
Learning Objectives
Learning objectives are stated at the beginning of each chapter. These learning
objectives serve as a preview of the information you are expected to learn in the
chapter. The comprehensive check questions are based on the objectives. By successfully
completing the NRTC, you indicate that you have met the objectives and have learned
the information. The learning objective are listed below.
Upon completion of this chapter, you should be able to do the following:
1. State, in terms of energy bands, the differences between a conductor,
an insulator, and a semiconductor.
2. Explain the electron and the hole flow theory in semiconductors and
how the semiconductor is affected by doping.
3. Define the term "diode" and give a brief description of its construction
and operation.
4. Explain how the diode can be used as a half-wave rectifier and as a
switch.
5. Identify the diode by its symbology, alphanumerical designation, and
color code.
6. List the precautions that must be taken when working with diodes and
describe the different ways to test them.
Introduction to SOLID-STATE DEVICES
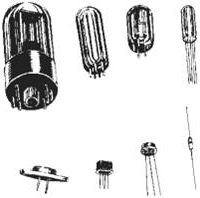
Figure 1-1. - Size comparisons of electron tubes and semiconductors.
As you recall from previous studies in this series, semiconductors have electrical
properties somewhere between those of insulators and conductors. The use of semiconductor
materials in electronic components is not new; some devices are as old as the electron
tube. Two of the most widely known semiconductors in use today are the JUNCTION
DIODE and Transistor. These semiconductors fall under a more general heading called
solid-state devices. a SOLID-STATE DEVICE is nothing more than an electronic device,
which operates by virtue of the movement of electrons within a solid piece of semiconductor
material.
Since the invention of the transistor, solid-state devices have been developed
and improved at an unbelievable rate. Great strides have been made in the manufacturing
techniques, and there is no foreseeable limit to the future of these devices. Solid-state
devices made from semiconductor materials offer compactness, efficiency, ruggedness,
and versatility. Consequently, these devices have invaded virtually every field
of science and industry. In addition to the junction diode and transistor, a whole
new family of related devices has been developed: the ZENER DIODE, LIGHT-EMITTING
DIODE, FIELD EFFECT Transistor, etc. One development that has dominated solid-state
technology, and probably has had a greater impact on the electronics industry than
either the electron tube or transistor, is the INTEGRATED Circuit. The integrated
circuit is a minute piece of semiconductor material that can produce complete electronic
circuit functions.
As the applications of solid-state devices mount, the need for knowledge of these
devices becomes increasingly important. Personnel in the Navy today will have to
understand solid-state devices if they are to become proficient in the repair and
maintenance of electronic equipment. Therefore, our objective in this module is
to provide a broad coverage of solid-state devices and, as a broad application,
power supplies. We will begin our discussion with some background information on
the development of the semiconductor. We will then proceed to the semiconductor
diode, the transistor, special devices and, finally, solid-state power supplies.
SEMICONDUCTOR DEVELOPMENT
Although the semiconductor was late in reaching its present development, its
story began long before the electron tube. Historically, we can go as far back as
1883 when Michael Faraday discovered that silver sulfide, a semiconductor, has a
negative temperature coefficient. The term negative temperature coefficient is just
another way of saying its resistance to electrical current flow decreases as temperature
increases. The opposite is true of the conductor. It has a positive temperature
coefficient. Because of this particular characteristic, semiconductors are used
extensively in power-measuring equipment.
Only 2 years later, another valuable characteristic was reported by Munk A. Rosenshold.
He found that certain materials have rectifying properties. Strange as it may seem,
his finding was given such little notice that it had to be rediscovered 39 years
later by F. Braun.
Toward the close of the 19th century, experimenters began to notice the peculiar
characteristics of the chemical element SELENIUM. They discovered that in addition
to its rectifying properties (the ability to convert ac into dc), selenium was also
light sensitive-its resistance decreased with an increase in light intensity. This
discovery eventually led to the invention of the photophone by Alexander Graham
Bell. The photophone, which converted variations of light into sound, was a predecessor
of the radio receiver; however, it wasn't until the actual birth of radio that selenium
was used to any extent. Today, selenium is an important and widely used semiconductor.
Many other materials were tried and tested for use in communications. SILICON
was found to be the most stable of the materials tested while GALENA, a crystalline
form of lead sulfide, was found the most sensitive for use in early radio receivers.
By 1915, Carl Beredicks discovered that GERMANIUM, another metallic element, also
had rectifying capabilities. Later, it became widely used in electronics for low-power,
low-frequency applications.
Although the semiconductor was known long before the electron tube was invented,
the semiconductor devices of that time could not match the performance of the tube.
Radio needed a device that could not only handle power and amplify but rectify and
detect a signal as well. Since tubes could do all these things, whereas semiconductor
devices of that day could not, the semiconductor soon lost out.
It wasn't until the beginning of World War II that interest was renewed in the
semiconductor. There was a dire need for a device that could work within the ultra-high
frequencies of radar. Electron tubes had interelectrode capacitances that were too
high to do the job. The point-contact semiconductor diode, on the other hand, had
a very low internal capacitance. Consequently, it filled the bill; it could be designed
to work within the ultra-high frequencies used in radar, whereas the electron tube
could not.
As radar took on greater importance and communication-electronic equipment became
more sophisticated, the demands for better solid-state devices mounted. The limitations
of the electron tube made necessary a quest for something new and different. An
amplifying device was needed that was smaller, lighter, more efficient, and capable
of handling extremely high frequencies. This was asking a
lot, but if progress was to be made, these requirements had to be met. a serious
study of semiconductor materials began in the early 1940's and has continued since.
In June 1948, a significant breakthrough took place in semiconductor development.
This was the discovery of Point-CONTACT Transistor. Here at last was a semiconductor
that could amplify. This discovery brought the semiconductor back into competition
with the electron tube. a year later, JUNCTION DIODES and Transistors were developed.
The junction transistor was found superior to the point-contact type in many respects.
By comparison, the junction transistor was more reliable, generated less noise,
and had higher power-handling ability than its point-contact brother. The junction
transistor became a rival of the electron tube in many uses previously uncontested.
Semiconductor diodes were not to be slighted. The initial work of Dr. Carl Zener
led to the development of ZENER DIODE, which is frequently used today to regulate
power supply voltages at precise levels. Considerably more interest in the solid-state
diode was generated when Dr. Leo Esaki, a Japanese scientist, fabricated a diode
that could amplify. The device, named the TUNNEL DIODE, has amazing gain and fast
switching capabilities. Although it is used in the conventional amplifying and oscillating
circuits, its primary use is in computer logic circuits.
Another breakthrough came in the late 1950's when it was discovered that semiconductor
materials could be combined and treated so that they functioned as an entire circuit
or subassembly rather than as a circuit component. Many names have been given to
this solid-circuit concept, such as INTEGRATED Circuits, MICROELECTRONICS, and MICROCircuitRY.
So as we see, in looking back, that the semiconductor is not something new, but
it has come a long way in a short time.
Q1. What is a solid-state device?
Q2. Define the term negative temperature coefficient.
SEMICONDUCTOR Applications
In the previous paragraphs, we mentioned just a few of the many different applications
of semiconductor devices. The use of these devices has become so widespread that
it would be impossible to list all their different applications. Instead, a broad
coverage of their specific application is presented.
Semiconductor devices are all around us. They can be found in just about every
commercial product we touch, from the family car to the pocket calculator. Semiconductor
devices are contained in television sets, portable radios, stereo equipment, and
much more.
Science and industry also rely heavily on semiconductor devices. Research laboratories use these devices in all sorts of electronic instruments to perform tests, measurements,
and numerous other experimental tasks. Industrial control systems (such as those used to manufacture automobiles) and automatic telephone exchanges also use semiconductors.
Even today heavy-duty versions of the solid- state rectifier diode are being use
to convert large amounts of power for electric railroads. Of the many different
applications for solid-state devices, space systems, computers, and data processing
equipment are some of the largest consumers.
The various types of modem military equipment are literally loaded with semiconductor
devices. Many radars, communication, and airborne equipment are transistorized.
Data display systems, data processing units, computers, and aircraft guidance-control
assemblies are also good examples of
electronic equipments that use semiconductor devices. All of the specific applications
of semiconductor devices would make a long impressive list. The fact is, semiconductors
are being used extensively in commercial products, industry, and the military.
SEMICONDUCTOR COMPETITION
It should not be difficult to conclude, from what you already know, that semiconductor
devices can and do perform all the conventional functions of rectification, amplification,
oscillation, timing, switching, and sensing. Simply stated, these devices perform
the same basic functions as the electron tube; but they perform more efficiently,
economically, and for a longer period of time. Therefore, it should be no surprise
to you to see these devices used in place of electron tubes. Keeping this in mind,
we see that it is only natural and logical to compare semiconductor devices with
electron tubes.
Physically, semiconductor devices are much smaller than tubes. You can see in
figure 1-1 that the difference is quite evident. This illustration shows some commonly used tube sizes alongside semiconductor devices of similar capabilities. The reduction
in size can be as great as 100:1 by weight and 1000:1 by volume. It is easy to see
that size reduction favors the semiconductor device. Therefore, whenever miniaturization
is required or is convenient, transistors are favored over tubes. Bear in mind,
however, that the extent of practical size reduction is a big factor; many things
must be considered. Miniature electron tubes, for example, may be preferred in certain
applications to transistors, thus keeping size reduction to a competitive area.
Power is also a two-sided story. For low-power applications, where efficiency
is a significant factor, semiconductors have a decided advantage. This is true mainly
because semiconductor devices perform very well with an extremely small amount of
power; in addition, they require no filaments or heaters as in the case of the electron
tube. For example, a computer operating with over 4000 solid-state devices may require
no more than 20 watts of power. However, the same number of tubes would require
several kilowatts of power.
For high-power applications, it is a different story - tubes have the upper hand.
The high-power tube has no equivalent in any semiconductor device. This is because
a tube can be designed to operate
with over a thousand volts applied to its plate whereas the maximum allowable
voltage for a transistor is limited to about 200 volts (usually 50 volts or less).
A tube can also handle thousands of watts of power. The maximum power output for
transistor generally ranges from 30 milliwatts to slightly over 100 watts.
When it comes to ruggedness and life expectancy, the tube is still in competition.
Design and functional requirements usually dictate the choice of device. However,
semiconductor devices are rugged and long-lived. They can be constructed to withstand
extreme vibration and mechanical shock. They have been known to withstand impacts
that would completely shatter an ordinary electron tube. Although some specially
designed tubes render extensive service, the life expectancy of transistors is better
than three to four times that of ordinary electronic tubes. There is no known failure
mechanism (such as an open filament in a tube) to limit the semiconductor's life.
However, semiconductor devices do have some limitations. They are usually affected
more by temperature, humidity, and radiation than tubes are.
Q3. Name three of the largest users of semiconductor devices.
Q4. State one requirement of an electron tube, which does not exist
for semiconductors, that makes the tube less efficient than the semiconductor.
SEMICONDUCTOR THEORY
To understand why solid-state devices function as they do, we will have to examine
closely the composition and nature of semiconductors. This entails theory that is
fundamental to the study of solid- state devices.
Rather than beginning with theory, let's first become reacquainted with some
of the basic information you studied earlier concerning matter and energy (NEETS,
Module 1).
ATOMIC STRUCTURE
The universe, as we know it today, is divided into two parts: matter and energy.
Matter, which is our main concern at this time, is anything that occupies space
and has weight. Rocks, water, air, automobiles, clothing, and even our own bodies
are good examples of matter. From this, we can conclude that matter may be found
in any one of three states: SOLIDS, LIQUIDS, and GASES. All matter is composed of
either an element or combination of elements. As you know, an element is a substance
that cannot be reduced to a simpler form by chemical means. Examples of elements
with which you are in contact everyday are iron, gold, silver, copper, and oxygen.
At present, there are over 100 known elements of which all matter is composed.
As we work our way down the size scale, we come to the atom, the smallest particle
into which an element can be broken down and still retain all its original properties.
The atoms of one element, however, differ from the atoms of all other elements.
Since there are over 100 known elements, there must be over 100 different atoms,
or a different atom for each element.
Now let us consider more than one element at a time. This brings us to the term
"compound." a compound is a chemical combination of two or more elements. Water,
table salt, ethyl alcohol, and ammonia are all examples of compounds. The smallest
part of a compound, which has all the characteristics of the compound, is the molecule.
Each molecule contains some of the atoms of each of the elements forming the compound.
Consider sugar, for example. Sugar in general terms is matter, since it occupies
space and has weight. It is also a compound because it consists of two or more elements.
Take a lump of sugar and crush
it into small particles; each of the particles still retains its original identifying
properties of sugar. The only thing that changed was the physical size of the sugar.
If we continue this subdividing process by grinding the sugar into a fine power,
the results are the same. Even dissolving sugar in water does not change its identifying
properties, in spite of the fact that the particles of sugar are now too small to
be seen even with a microscope. Eventually, we end up with a quantity of sugar that
cannot be further divided without its ceasing to be sugar. This quantity is known
as a molecule of sugar. If the molecule is further divided, it is found to consist
of three simpler kinds of matter: carbon, hydrogen, and oxygen. These simpler forms
are called elements. Therefore, since elements consist of atoms, then a molecule
of sugar is made up of atoms of carbon, hydrogen, and oxygen.
As we investigate the atom, we find that it is basically composed of electrons,
protons, and neutrons. Furthermore, the electrons, protons, and neutrons of one
element are identical to those of any other element. There are different kinds of
elements because the number and the arrangement of electrons and protons are different
for each element.
The electron carries a small negative charge of electricity. The proton carries
a positive charge of electricity equal and opposite to the charge of the electron.
Scientists have measured the mass and size of the electron and proton, and they
know how much charge each possesses. Both the electron and proton have the same
quantity of charge, although the mass of the proton is approximately 1,827 times
that of the electron. In some atoms there exists a neutral particle called a neutron.
The neutron has a mass approximately equal to that of a proton, but it has no electrical
charge.
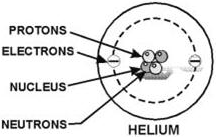
Figure 1-2. - The composition of a simple helium atom.
According to theory, the electrons, protons, and neutrons of the atoms are thought
to be arranged in a manner similar to a miniature solar system. Notice the helium
atom in figure 1-2. Two protons and two neutrons form the heavy nucleus with a positive
charge around which two very light electrons revolve. The path each electron takes
around the nucleus is called an orbit. The electrons are continuously being acted
upon in their orbits by the force of attraction of the nucleus. To maintain an orbit
around the nucleus, the electrons travel at a speed that produces a counterforce
equal to the attraction force of the nucleus. Just as energy is required to move
a space vehicle away from the earth, energy is also required to move an electron
away from the nucleus. Like a space vehicle, the electron is said to be at a higher
energy level when it travels a larger orbit. Scientific experiments have shown that
the electron requires a certain amount of energy to stay in orbit. This quantity
is called the electron's energy level. By virtue of just its motion alone, the electron
contains kinetic energy. Because of its position, it also contains potential energy.
The total energy contained by an electron (kinetic energy plus potential energy)
is the main factor that determines the radius of the electron's orbit. For an electron
to remain in this orbit, it must neither gain nor lose energy.
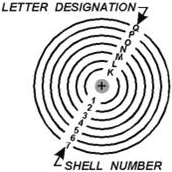
Figure 1-3. - Shell designation.
The orbiting electrons do not follow random paths, instead they are confined
to definite energy levels. Visualize these levels as shells with each successive
shell being spaced a greater distance from the nucleus. The shells, and the number
of electrons required to fill them, may be predicted by using Pauli's exclusion
principle. Simply stated, this principle specifies that each shell will contain
a maximum of 2n2 electrons, where n corresponds to the shell number starting with
the one closest to the nucleus. By this principle, the second shell, for example,
would contain 2(2) 2 or 8 electrons when full.
In addition to being numbered, the shells are also given letter designations
starting with the shell closest to the nucleus and progressing outward as shown
in figure 1-3. The shells are considered to be full, or complete, when they contain
the following quantities of electrons: 2 in the K(1st) shell, 8 in the L(2nd) shell,
18 in the M(3rd) shell, and so on, in accordance with the exclusion principle. Each
of these shells is a major shell and can be divided into subshells, of which there
are four, labeled s, p, d, and f. Like the major shells, the subshells are also
limited as to the number of electrons they contain. Thus, the "s" subshell is
complete when it contains 2 electrons, the "p" subshell when it contains 6, the
"d" subshell when it contains 10, and the "f" subshell when it contains 14 electrons.
Inasmuch as the K shell can contain no more than 2 electrons, it must have only
one subshell, the s subshell. The M shell is composed of three subshells: s, p,
and d. If the electrons in the s, p, and d subshells are added together, their total
is found to be 18, the exact number required to fill the M shell. Notice the electron
configuration of copper illustrated in figure 1-4. The copper atom contains 29 electrons,
which completely fill the first three shells and subshells, leaving one electron
in the "s" subshell of the N shell. a list of all the other known elements, with
the number of electrons in each atom, is contained in the PERIODIC TABLE of ELEMENTS.
The periodic table of elements is included in appendix 2.
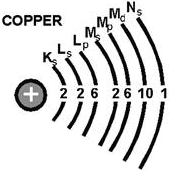
Figure 1-4. - Copper atom.
Valence is an atom's ability to combine with other atoms. The number of electrons
in the outermost shell of an atom determines its valence. For this reason, the outer
shell of an atom is called VALENCE SHELL, and the electrons contained in this shell
are called VALENCE ELECTRONS. The valence of an atom determines its ability to gain
or lose an electron, which in turn determines the chemical and electrical properties
of the atom. An atom that is lacking only one or two electrons from its outer shell
will easily gain electrons to complete its shell, but a large amount of energy is
required to free any of its electrons. An atom having a relatively small number
of electrons in its outer shell in comparison to the number of electrons required
to fill the shell will easily lose these valence electrons. The valence shell always
refers to the outermost shell.
Q5. Define matter and list its three different states.
Q6. What is the smallest particle into which an element can be broken
down and still retain all its original properties?
Q7. What are the three particles that comprise an atom and state
the type of charge they hold?
Q8. What is the outer shell of an atom called?
ENERGY BandS
Now that you have become reacquainted with matter and energy, we will continue
our discussion with electron behavior.
As stated earlier, orbiting electrons contain energy and are confined to definite
energy levels. The various shells in an atom represent these levels. Therefore,
to move an electron from a lower shell to a higher shell a certain amount of energy
is required. This energy can be in the form of electric fields, heat, light, and
even bombardment by other particles. Failure to provide enough energy to the electron,
even if the energy supplied is just short of the required amount, will cause it
to remain at its present energy level. Supplying more energy than is needed will
only cause the electron to move to the next higher shell and the remaining energy
will be wasted. In simple terms, energy is required in definite units to move electrons
from one shell to the next higher shell. These units are called QUANTA (for example
1, 2, or 3 quanta).
Electrons can also lose energy as well as receive it. When an electron loses
energy, it moves to a lower shell. The lost energy, in some cases, appears as heat.
If a sufficient amount of energy is absorbed by an electron, it is possible for
that electron to be completely removed from the influence of the atom. This is called
IONIZATION. When an atom loses electrons or gains electrons in this process of electron
exchange, it is said to be ionized. For ionization to take place, there must be
a transfer of energy that results in a change in the internal energy of the atom.
An atom having more than its normal amount of electrons acquires a negative charge,
and is called a Negative ION. The atom that gives up some of its normal electrons
is left with fewer negative charges than positive charges and is called a Positive
ION. Thus, we can define ionization as the process by which an atom loses or gains
electrons.
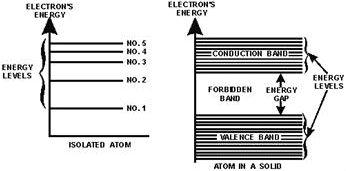
Figure 1-5. - The energy arrangement in atoms.
Up to this point in our discussion, we have spoken only of isolated atoms. When
atoms are spaced far enough apart, as in a gas, they have very little influence
upon each other, and are very much like lone atoms. But atoms within a solid have
a marked effect upon each other. The forces that bind these atoms together greatly
modify the behavior of the other electrons. One consequence of this close proximity
of atoms is to cause the individual energy levels of an atom to break up and form
bands of energy. Discrete (separate and complete) energy levels still exist within
these energy bands, but there are many more energy levels than there were with the
isolated atom. In some cases, energy levels will have disappeared. Figure 1-5 shows
the difference in the energy arrangement between an isolated atom and the atom in
a solid. Notice that the isolated atom (such as in gas) has energy levels, whereas
the atom in a solid has energy levels grouped into ENERGY BandS.
The upper band in the solid lines in figure 1-5 is called the CONDUCTION Band
because electrons in this band are easily removed by the application of external
electric fields. Materials that have a large number of electrons in the conduction
band act as good conductors of electricity.
Below the conduction band is the forBIDDEN Band or energy gap. Electrons are
never found in this band, but may travel back and forth through it, provided they
do not come to rest in the band.
The last band or VALENCE Band is composed of a series of energy levels containing
valence electrons. Electrons in this band are more tightly bound to the individual
atom than the electrons in the conduction band. However, the electrons in the valence
band can still be moved to the conduction band with the application of energy, usually
thermal energy. There are more bands below the valence band, but they are not important
to the understanding of semiconductor theory and will not be discussed.
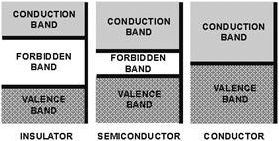
Figure 1-6. - Energy level diagram.
The concept of energy bands is particularly important in classifying materials
as conductors, semiconductors, and insulators. An electron can exist in either of
two energy bands, the conduction band or the valence band. All that is necessary
to move an electron from the valence band to the conduction band so it can be used
for electric current, is enough energy to carry the electron through the forbidden
band. The width of the forbidden band or the separation between the conduction and
valence bands determines whether a substance is an insulator, semiconductor, or
conductor. Figure 1-6 uses energy level diagrams to show the difference between
insulators, semiconductors, and conductors.
The energy diagram for the insulator shows the insulator with a very wide energy
gap. The wider this gap, the greater the amount of energy required to move the electron
from the valence band to the conduction band. Therefore, an insulator requires a
large amount of energy to obtain a small amount of current. The insulator "insulates"
because of the wide forbidden band or energy gap.
The semiconductor, on the other hand, has a smaller forbidden band and requires
less energy to move an electron from the valence band to the conduction band. Therefore,
for a certain amount of applied voltage, more current will flow in the semiconductor
than in the insulator.
The last energy level diagram in figure 1-6 is that of a conductor. Notice, there
is no forbidden band or energy gap and the valence and conduction bands overlap.
With no energy gap, it takes a small amount of energy to move electrons into the
conduction band; consequently, conductors pass electrons very easily.
Q9. What term is used to describe the definite discrete amounts of
energy required to move an electron from a low shell to a higher shell?
Q10. What is a negative ion?
Q11. What is the main difference in the energy arrangement between
an isolated atom and the atom in a solid?
Q12. What determines, in terms of energy bands, whether a substance
is a good insulator, semiconductor, or conductor?
COVALENT BONDING
The chemical activity of an atom is determined by the number of electrons in
its valence shell. When the valence shell is complete, the atom is stable and shows
little tendency to combine with other atoms to form solids. Only atoms that possess
eight valence electrons have a complete outer shell. These atoms are
1-10 <next>
- |
Matter, Energy,
and Direct Current |
- |
Alternating Current and Transformers |
- |
Circuit Protection, Control, and Measurement |
- |
Electrical Conductors, Wiring Techniques,
and Schematic Reading |
- |
Generators and Motors |
- |
Electronic Emission, Tubes, and Power Supplies |
- |
Solid-State Devices and Power Supplies |
- |
Amplifiers |
- |
Wave-Generation and Wave-Shaping Circuits |
- |
Wave Propagation, Transmission Lines, and
Antennas |
- |
Microwave Principles |
- |
Modulation Principles |
- |
Introduction to Number Systems and Logic Circuits |
- |
- Introduction to Microelectronics |
- |
Principles of Synchros, Servos, and Gyros |
- |
Introduction to Test Equipment |
- |
Radio-Frequency Communications Principles |
- |
Radar Principles |
- |
The Technician's Handbook, Master Glossary |
- |
Test Methods and Practices |
- |
Introduction to Digital Computers |
- |
Magnetic Recording |
- |
Introduction to Fiber Optics |
Note: Navy Electricity and Electronics Training
Series (NEETS) content is U.S. Navy property in the public domain. |
|