January 1958 Radio-Electronics
[Table
of Contents]
Wax nostalgic about and learn from the history of early electronics.
See articles from Radio-Electronics,
published 1930-1988. All copyrights hereby acknowledged.
|
Like so many things
in life that we take for granted - aspirin, automatic clothes washers, drill
motors and bits, eyeglasses, rifles, bicycles, transistors, to name a few -
we rarely think about the effort that went behind the end product that is now
enjoyed. Even relatively simple devices like scissors are the result of someone
saying to himself or herself, "Self, I need something to make cutting fabric
and paper and hair simpler and neater, so what might that thing look like?"
Then, after making a working prototype, improvements are made based on empirical
testing from usage, improvements are made in the form factor, materials, size,
etc., until evolution results in what can be purchased today. If you have ever
been in a product design cycle, either privately or corporately, then you know
the process well. A concept as sophisticated as developing television is much
more involved than developing a pair of scissors, but the fundamentals are the
same. Implementing color TV for commercial broadcast had the dual difficulty
of not just producing affordable and reliable color images, but also being backwards
compatible with black and white (B&W) broadcasts and TV sets already in
place. Adding stereo sound to the original monaural FM radio broadcasts had
the same requirement. This article from a 1958 edition of Radio-Electronics
does a superb job of describing the work that went into implementing color television.
You might be as surprised as I was to learn how much new science was required
in the realm of human vision and color perception in order to design a high
quality transmission and presentation. Prior to color TV, most color perception
research went into reflected light (paint, ink, crayons, etc.) whereas for the
first time in human history it was necessary to determine the peculiarities
of emitted light from a light source (cathode ray tube).
The Strange World of Color Vision

The Cover
Our cover this month is an abstraction based on a suggestion by Mr. Middleton
to illustrate this article. The two odd-looking discs are used to produce
sensations of color from black and white elements only. (See Fig. 6.)
The bar slanting across the bottom is, of course, the color spectrum as most
of us know it, and the upright panel is the color spectrum of a color-anomalous
or "color-blind" person, whose world of color is based on stimuli from two
instead of three primaries. The floating colored dots or bubbles, chosen to
produce a random display of color, are actually a nighttime color photograph
of colored lights on a Ferris Wheel deliberately shot out of focus. The picture
was taken by Jay Maisel.
|

Fig. 1 - As wavelength of electromagnetic waves is
raised from 380 to 780 milli-microns, we see in succession all the colors
of the rainbow.
|

Fig. 2 - The standard color map or chromaticity diagram.
All colors are arranged around white as an optical center.
|
By Robert G. Middleton Television Consultant
Color is by no means simple - it is often incomprehensible and always
tricky; but its apparent inconsistencies make compatible color television possible.
Children and simple folk suppose that the colors they see really exist in
nature and scoff at the idea that colors exist only in the mind.
Physicists explain that the colors we see correspond to waves of electromagnetic
energy from 4 to 8 X 1014 cycles per second. Electromagnetic waves
used in radio and television transmission have longer wavelengths.
Of course, light waves are not color, any more than radio waves are color.
Physicists do not attempt to explain further and physiologists cannot. Psychologists
are baffled and philosophers offer various theories which cannot be proved or
disproved.
In spite of the unsatisfactory state of our knowledge concerning color vision,
many interesting laws have been discovered, upon which the technology of color
television rests. Some of these laws are well known while others are familiar
only to specialists.
Trichromatic Vision
Of all our body's organs, the eye is most remarkable. Loss of hearing is
a personal tragedy but loss of sight is a calamity - our eyes provide us with
more information concerning the external world than any other organ.
It was once supposed that the eye is a frequency-sensitive organ because
we see various colors when electromagnetic waves of various frequencies enter
the eye. As shown in Fig. 1, a wavelength of 475 mμ (millimicrons,
mμ) causes us to see blue, 520 mμ green, 578 mμ yellow and 700 mμ
to see red.
Fig. 1 shows in a limited manner the information given in Fig. 2.
The chromaticity diagram (Fig. 2) shows around its border the wavelengths
of light corresponding to the common colors. Note that there are colors along
the base of the diagram to which no single wavelength of light corresponds.
This is a rather unexpected fact which is discussed later in the article.

Fig. 3 - Red and green combine to form yellow; red
and blue combine to form magenta; green and blue combine to form cyan.
Fig. 4 - Red, green and blue combine to form white.
Fig. 5. - Each color signal voltage has a phase as
well as an amplitude.
Fig. 6 - Black and white patterns which generate the
sensation of colors when rotated.
Note: I printed the patterns of Fig. 6 and glued a round toothpick
through the center of each. (a) looked sort of bluish and (b) looked sort
of reddish, but there was no clearly discernable color.
|
In view of such experimental data, it is reasonable to conclude that each color
we see has a corresponding frequency or wavelength. However, there are difficulties
which make this conclusion unacceptable.
When we mix red light with green light, we do not see either of these two
colors. Instead we see a new color: yellow. On this basis, we must abandon the
definition that yellow corresponds to a wavelength of 578 mμ, since yellow
is also produced by a combination of two other wavelengths.
In fact, investigation has shown that the many thousands of colors which
we see can be obtained by mixing only three colored lights - red, green and
blue - in various proportions. Color television operates upon this law, the
law of trichromatic vision. Fig. 3 illustrates how the primary colors of
red, green and blue combine by pairs to form the complementary colors of yellow,
cyan and magenta. Fig. 4 shows how the three primary colors combine to
form white.
To obtain compatible operation of black-and-white and color TV receivers,
wavelengths of light are transmitted as various phase angles of a color subcarrier.
This is shown in Fig. 5. Burst is taken as the reference frequency. Red,
which has a wavelength of 700 mμ, is transmitted as a phase angle of 76.5°.
Magenta, which is a combination of 700 mμ and 475 mμ, is transmitted
as a phase angle of 119°.
The colors seen in the spectrum of a prism are 100% saturated. They are pure
colors. Saturated colors are vivid. Desaturated colors are pale - they have
a pastel shade. The wavelength of a desaturated color is the same as the wavelength
of the same saturated color. However, white light is mixed with a saturated
color to make a desaturated color. In Fig. 5, the relative voltages of
the saturated colors are shown by the lengths of the vectors. Now, if we shorten
the length of the red vector to half that shown in Fig. 5, we transmit
pink - a desaturated red.
It is no mystery that hues are transmitted in terms of phase, and that saturations
are transmitted in terms of voltage. These values are easily calculated throughout.
We encounter the unknown only when we attempt to understand how a color such
as yellow is seen when the eye is viewing a mixture of red and green lights.
Perhaps we shall never know.
Producing Color
White is a mixture of red, green and blue. Black is the absence of visible
electromagnetic wave energy. As white can be produced from colors, conversely,
colors can be produced by suitable arrangements of black and white. For example,
when we mount the disk shown in Fig. 6-a on the shaft of a variable-speed
motor, we see an arc of color in the rotating pattern. As the speed of the motor
is varied, the hue of the color changes accordingly. A disk which produces red
and yellow is shown in Fig. 6-b.
It is thought that there may be three types of color receptors in the retina
of the eye, with peak responses to wavelengths in the regions of red, green
and blue. These color receptors are not sharply tuned but have overlapping responses
or considerable bandwidth. Furthermore, when these color receptors are simultaneously
energized by white light which is then suddenly stopped, the response of the
color receptors does not fall to zero at once, but requires a small time interval
to decay to zero.

Fig. 7-a - Splitting white light
into a color spectrum; b - recombination of color spectrum produces white
light.

Fig. 8 - 0ptical filters use
resonant electrical circuits which are provided by nature in the electronic
orbits of the atoms comprising the filter glass.
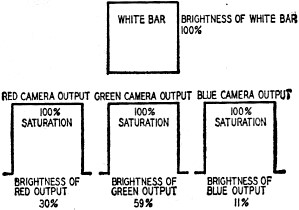
Fig. 9 - The brightness of a
white bar, like that of a color bar, is equal to the sum of the brightness
of its components.
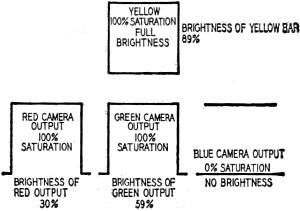
Fig. 10 - The brightness of a
color is equal to the sum of the brightness of its components.

Fig. 11 - Color is characterized
by three quantities: brightness, hue and saturation. Color brightness is determined
only by Y-voltage value and is not affected by chrominance voltage.

Fig. 12 - The chrominance voltages
produced by scanning 25%, 50%, 75% and 100% saturated red. Length of chrominance
vector is directly proportional to color saturation. Its phase does not change
with change in saturation.

Fig. 13 - Compatibility of color-TV
and black-and-white TV requires that the Y signal as used in black-and-white
transmission be unaltered.
|
Because the color receptors have differing decay times, a residual unbalance
of response occurs from the receptors during the decay time so that we see color
in the black-and-white whirling pattern, which attacks the eye with sudden changes
from black to white and vice versa. The disk should be rotated counterclockwise
at a speed on the borderline of persistence of vision, and the level of daylight
or artificial light adjusted to produce the maximum intensity of color in the
whirling pattern. Thin, fairly intense red rings, blue tails and yellow fields
will appear under suitable conditions.
The known facts of "color blindness" also support the theory of three color
receptors in the retina, responding in the regions of red, green and blue.
Test charts are available, which reveal the presence of various types of
color blindness in afflicted persons. Some persons are completely color-blind,
but still get about quite handily, since black-and-white vision remains (provided
by the rods in the retina) although the color receptors (cones) are incapable
of response.
All of us are color-blind in dim light. If you walk out of a lighted room
into a dimly lit hall and look at a color chart, you will find that all the
colors appear to be in shades of gray.
We are also color-blind to very small patches of color and semi-color-blind
to somewhat larger patches. All colors seem to drift into orange or cyan hues
as a patch of the color is reduced in size. This is the I axis of color television
and is the basis of wide-band color transmission.
Development of the Eye
In an embryonic infant, the retina develops as an outgrowth of the fore-brain.
However, the function of vision is located in the hind-brain and persons who
suffer injury to the hind-brain are as blind as if their eyes had been put out.
If a person has the hind-brain intact, but his eyes have been injured, he
may still see flashes of light and colors as a result of mechanical stimulation
of the exposed ends of the optic nerves. A somewhat similar response is observed
by normal persons. If the eyelids are closed and pressure is applied to the
edge of the eyeball, rings of yellowish light are seen.
How the sensation of light is produced in the mind when the optic nerves
are energized is not known. As far as color television is concerned, it is essential
only that we know the laws of color mixture whereby any desired color can be
synthesized by a suitable mixture of the three primary colors.
Fig. 7-a shows how a prism can decompose white light into a spectrum
of its color components. Fig. 7-b shows how the color spectrum can be recombined
into white light again. This is not a very striking demonstration. However,
we find that we can remove large regions of the spectrum, leaving only the colors
at the extreme ends and a color at the middle, and still obtain white on re-composition.
This robbed white is quite undistinguishable by the human eye from the first
white.
If we were unable to rob the spectrum in this manner, color television as
we know it today would be impossible. It is hard enough to transmit three primary-color
signals in a 6-mc channel containing a black-and-white signal and sound signal
without contending with individual signals for tens of thousands of particular
hues.
Robbing the Color Spectrum
A rainbow spectrum of light is conveniently robbed by use of filters, as
depicted in Fig. 8. Optical filters operate like electric or electronic
filters, except that the resonant circuits utilized are contained in the electronic
orbits of the atoms in the filter.
All matter is an arrangement of electrical forces or fields. The electrons
comprising a substance have a certain frequency of rotation about the nucleus
of the atom and are coupled to external space just as an antenna is coupled
to space. Incident electromagnetic energy can be absorbed by electrons in certain
orbits, just as a tuned circuit absorbs electromagnetic energy from an antenna.
Thus, an optical filter is an electronic wavetrap, of atomic dimensions,
which is tuned to the frequency of the electromagnetic energy recognized by
us as a given color. There is no basic difference between an optical filter
and a wave trap except that the wavelengths used in the optical filter are shorter.
Color can be specified on a technical basis in terms of brightness, hue and
saturation. Fig. 9 shows that the brightness of a white bar is equal to
the sum of the brightnesses of its components (red, green and blue). The brightness
of red, as seen on a black-and-white picture tube, is 30%; the brightness of
green is 59%; the brightness of blue is 11 % - hence, the brightness of white
is 100%.
Fig. 10 shows that the brightness of a color is equal to the sum of
the brightnesses of its components. Yellow has a brightness, as seen on a black-and-white
picture tube, of 89%. Yellow is comprised of green which has a brightness of
59% and of red which has a brightness of 30% - hence, the brightness of yellow
is the sum of these brightnesses, or 89%.
The brightness of a color, as transmitted from a color TV station, is given
by the level of the Y (black-and-white) signal component, as shown in Fig. 11.
To this brightness signal level is added a 3.58-mc chroma signal. The chroma
signal specifies hue and saturation. Fig. 12 shows how the voltage of the
chroma signal specifies the saturation (vividness) of the color. We have already
seen how the phase of the chroma signal specifies color (hue).
This particular (NTSC) signal arrangement has been established to provide
compatibility in black-and-white and color TV reception. Fig. 13 shows
how a succession of red, green and blue bars is transmitted as a series of video
levels at 30%, 59% and 11%. This is the only part of the complete color signal
which is seen by a black-and-white TV receiver.
The chroma signal has a relatively high frequency (3.579545 mc) which is
largely filtered out in the if and video amplifier of a black-and-white TV receiver.
In a color TV receiver, however, the chroma signal component is not rejected,
but is processed through the chroma circuits of the color receiver.
The color receiver responds to the phase of the chroma signal by displaying
a corresponding hue; it responds to the voltage of the chroma signal by displaying
a corresponding color saturation. This is how we get compatibility.
Color and Monochrome (B&W) Television
Articles
Posted July 29, 2020 (updated from original post on 1/7/2014)
|