Module 18 - Radar PrinciplesPages
i,
1-1, 1-11,
1-21,
1-31,
1-41,
2-1,
2-11,
2-21,
2-31,
2-41,
3-1,
3-11,
3-21,
4-1,
4-11,
4-21,
AI-1,
AII-1,
Index-1 to 3
radar. a
two-dimensional search radar, however, does use a single-lobe that is scanned in a 360-degree pattern because
automatic tracking circuits are not normally used in 2D radars. Single-lobe scanning is unsuitable for use
as a tracking radar for several reasons. For example, let's assume that a target is somewhere on the lobe axis and
the receiver is detecting signals reflected from the target. If these reflected signals begin to decrease in
strength, the target likely has flown off the lobe axis. In this case, the beam must be moved to continue
tracking. The beam might be moved by an operator tracking the target with an optical sight, but such tracking is
slow, inaccurate, and limited by conditions of visibility. An automatic tracking system would require that the
beam SCAN, or search, the target area in such a case. AAgain, assume that a missile is riding (following) the
axis of a single beam. The strength of the signals it receives (by means of a radar receiver in the missile) will
gradually decrease as its distance from the transmitter increases. If the signal strength decreases suddenly, the
missile will know, from built-in detection circuitry, that it is no longer on the axis of the lobe. But it will
not know which way to turn to get back on the axis. a simple beam does not contain enough information for missile
guidance. Methods of Beam Scanning The two basic methods of beam scanning are
MECHANICAL and ELECTRONIC. In mechanical scanning, the beam can be moved in various ways: (1) The entire antenna
can be moved in the desired pattern; (2) the energy feed source can be moved relative to a fixed reflector; or (3)
the reflector can be moved relative to a fixed source. In electronic scanning, the beam is effectively moved by
such means as (1) switching between a set of feeder sources, (2) varying the phasing between elements in a
multielement array, or (3) comparing the amplitude and phase differences between signals received by a
multielement array. a combination of mechanical and electronic scanning is also used in some antenna systems.
MECHANICAL SCANNING. - The most common type of mechanical scanning is the rotation of the
antenna through 360 degrees to obtain azimuth coverage. Most search radar sets use this method. a common form of
scanning for target tracking or missile beam-rider systems is CONICAL (cone-like) SCANNING. This is generally
accomplished mechanically by NUTATING the RF feed point. Nutation is difficult to describe in words but
easy to demonstrate. Hold a pencil in two hands. While holding the eraser end as still as possible, swing the
point in a circular motion. This motion of the pencil is referred to as nutation; the pencil point corresponds to
the open, or transmitting, end of the waveguide antenna. The important fact to remember is that polarization of
the beam is not changed during the scanning cycle. This means that the axis of the moving feeder does not change
either horizontal or vertical orientation while the feeder is moving. You might compare the feeder movement to
that of a ferris wheel; that is, the vertical orientation of each seat remains the same regardless of the position
of the wheel. Recall that a waveguide is a metal pipe, usually rectangular in cross section, used to
conduct the f energy from the transmitter to the antenna. The open end of the waveguide faces the concave side of
the reflector and the RF energy it emits is bounced from the reflector surface. A conical scan can be
generated by nutation of the waveguide. In this process the axis of the waveguide itself is moved through a small
conical pattern. In an actual installation of a nutating waveguide, the three-dimensional movement is fast and of
small amplitude. To an observer, the waveguide appears merely to be vibrating slightly. By movement of
either the waveguide or the antenna, you can generate a conical scan pattern, as shown in figure 1-17. The axis of
the radar lobe is made to sweep out a cone in space; the apex of this cone is, of course, at the radar transmitter
antenna or reflector. At any given distance from the antenna, 1-21
the path of the lobe axis is a circle. Within the useful range of the beam, the inner edge of the lobe
always overlaps the axis of scan. 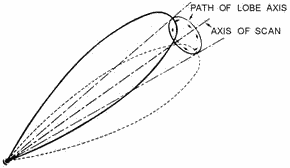
Figure 1-17. - Conical scanning. Now assume that we use a conically scanned beam for target tracking. If the target is on the scan axis, the
strength of the reflected signals remains constant (or changes gradually as the range changes). But if the target
is slightly off the axis, the amplitude of the reflected signals will change at the scan rate. For example, if the
target is to the left of the scan axis, as shown in figure 1-18, the reflected signals will be of maximum strength
as the lobe sweeps through the left part of its cone; the signals will quickly decrease to a minimum as the lobe
sweeps through the right part. Information on the instantaneous position of the beam, relative to the scan axis,
and on the strength of the reflected signals is fed to a computer. Such a computer in the radar system is referred
to as the angle-tracking or angle-servo circuit (also angle-error detector). If the target moves off the scan
axis, the computer instantly determines the direction and amount of antenna movement required to continue
tracking. The computer output is used to control servomechanisms that move the antenna. In this way, the target is
tracked accurately and automatically.
1-22
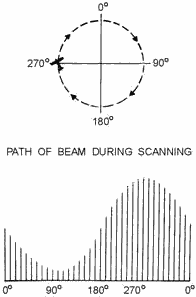
Figure 1-18. - Reflected signal strength.
Q20. What is the simplest type of scanning? Q21. How does the operator of a single-lobe scanning
system determine when the target moves off the lobe axis? Q22. What are the two basic methods of
scanning?
Q23. Rotation of an rf-feed source to produce a conical scan pattern is identified by what term?
ELECTRONIC SCANNING. - Electronic scanning can accomplish lobe motion more rapidly than, and
without the inherent maintenance disadvantages of, the mechanical systems. Because electronic scanning cannot
generally cover as large an area of space, it is sometimes combined with mechanical scanning in particular
applications. With MONOPULSE (SIMULTANEOUS) LOBING, all range, bearing, and elevation-angle information of a
target is obtained from a single pulse. Monopulse scanning is used in fire-control tracking radars. For target
tracking, the radar discussed here produces a narrow circular beam of pulsed-rf energy at a high pulse-repetition
rate. Each pulse is divided into four signals which are equal both in amplitude and phase. These four signals are
radiated at the same time from each of four feedhorns that are grouped in a cluster. The resulting radiated energy
is focused into a beam by a microwave lens. Energy reflected from targets is refocused by the lens back into the
feedhorns. The total amount of the energy received by each horn varies, depending on the position of the target
relative to the beam axis. This is illustrated in figure 1-19 for four targets at different positions with respect
to the beam axis. Note that a phase inversion takes place at the microwave lens similar to the image inversion
that takes place in an optical system. 1-23
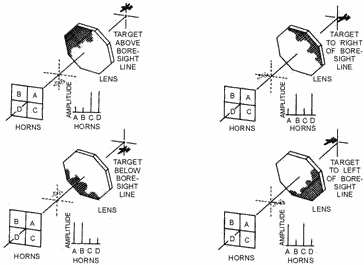
Figure 1-19. - Monopulse scanning. The amplitude of returned signals received by each horn is continuously compared with those received in the
other horns. Error signals are generated which indicate the relative position of the target with respect to the
axis of the beam. Angle servo circuits receive these error signals and correct the position of the radar beam to
keep the beam axis on target. The TRAVERSE (BEARING) Signal is made up of signals from horn a added to C
and from horn B added to D. By waveguide design, the sum of B and D is made 180 degrees out of phase with the sum
of a and C. These two are combined and the traverse signal is the difference of (A + C) - (B + D). Since the horns
are positioned as shown in figure 1-19, the relative amplitudes of the horn signals give an indication of the
magnitude of the traverse error. The elevation signal consists of the signals from horns C and D added 180 degrees
out of phase with horns a and B [(A + B) - (C + D)]. The sum, or range, signal is composed of signals from all
four feedhorns added together in phase. It provides a reference from which target direction from the center of the
beam axis is measured. The range signal is also used as a phase reference for the traverse and elevation-error
signals. The traverse and elevation error signals are compared in the radar receiver with the range or
reference signal. The output of the receiver may be either positive or negative pulses; the amplitudes of the
pulses are proportional to the angle between the beam axis and a line drawn to the target. The polarities of the
output pulses indicate whether the target is above or below, to the right or to the left of the beam axis. Of
course, if the target is directly on the line of sight, the output of the receiver is zero and no angle-tracking
error is produced. An important advantage of a monopulse-tracking radar over radar using conical scan is
that the instantaneous angular measurements are not subject to errors caused by target SCINTILLATION.
Scintillation can occur as the target maneuvers or moves and the radar pulses bounce off different areas of the
target. This causes random reflectivity and may lead to tracking errors. Monopulse tracking radar is not subject
to this type of error because each pulse provides an angular measurement without regard to the
1-24
rest of the pulse train; no such cross-section fluctuations can affect the measurement. An additional
advantage of monopulse tracking is that no mechanical action is required. ELECTRONIC SCANNING used in
search radar systems was explained in general terms earlier in this chapter during the discussion of elevation
coverage. This type of electronic scanning is often called Frequency SCANNING. An in-depth explanation of
frequency scanning theory can be found in the fire control technician rate training manuals. Radar Transmission Methods Radar systems are normally divided into operational categories based on energy transmission methods. Up to
this point, we have mentioned only the pulse method of transmission to illustrate basic radar concepts. Although
the pulse method is the most common method of transmitting radar energy, two other methods are sometimes used in
special applications. These are the continuous-wave (cw) method and the frequency modulation (FM) method. All
three basic transmission methods are often further subdivided to designate specific variations or combinations.
CONTINUOUS-WAVE METHOD
When radio-frequency energy transmitted from a fixed point continuously strikes an object that is either
moving toward or away from the source of the energy, the frequency of the reflected energy is changed. This shift
in frequency is known as the DOPPLER EFFECT. The difference in frequency between the transmitted and reflected
energy indicates both the presence and the speed of a moving target. Doppler Effect A
common example of the Doppler effect in action is the changing pitch of the whistle of an approaching train. The
whistle appears to change pitch from a high tone, as the train approaches, to a lower tone as it moves away from
the observer. As the train approaches, an apparent increase in frequency (an increase in pitch) is heard; as the
train moves away, an apparent decrease in frequency (a decrease in pitch) is heard. This pitch variation is known
as the Doppler effect. Let's examine the reason for this apparent change in pitch. Assume that the transmitter emits an audio signal
at a frequency of 60 hertz and that the transmitter is traveling at a velocity of 360 feet per second (fps). At
the end of 1 second, the transmitter will have moved from point P to point P1 as shown in view a of figure 1-20.
The total distance from point P to the observer is 1,080 feet. The velocity of sound is 1,080 feet per second;
thus, a sound emitted at point P will reach the observer in 1 second. To find the wavelength of this transmitted
signal, you divide the velocity of the signal (1,080 fps) by the frequency (60 hertz). The result is 18 feet, as
shown below:

1-25

Figure 1-20. - Transmitter moving relative to an observer. In 1 second the transmitter moves 360 feet and transmits 60 hertz. At the end of 1 second, the first cycle of
the transmitted signal reaches the observer, just as the sixtieth cycle is leaving the transmitter at point P1.
Under these conditions the 60 hertz emitted is located between the observer and point P1. Notice that this
distance is only 720 feet (1,080 minus 360). The 60 hertz is spread over the distance from point P1 to the
observer and has a wavelength of just 12 feet (720 divided by 60). To find the new frequency, use the following
formula:
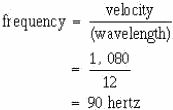
The original frequency, 60 hertz, has changed to an apparent frequency of 90 hertz. This new frequency only
applies to the observer. Notice that the Doppler frequency variation is directly proportional to the velocity of
the approaching transmitter. The faster the transmitter moves toward the observer, the greater the number of waves
that will be crowded into the space between the transmitter and the observer. Suppose the transmitter were
stationary and the observer moving. When approaching the transmitter, the observer would encounter waves per unit
of time. As a result, the observer would hear a higher pitch than the transmitter would actually emit. If
the transmitter were traveling away from the observer, as shown in view B of figure 1-20, the first cycle would
leave the transmitter at point P and the sixtieth at point P2. The first cycle would reach the observer when the
transmitter reached P2. You would then have 60 cycles stretched out over 1,080 plus 360 feet, a total of 1,440
feet. The wavelength of these 60 hertz is 1,440/60, or 24 feet. The apparent frequency is 1,080 divided by 24, or
45 hertz. Uses of CW Doppler System
The continuous-wave, or Doppler, system is used in several ways. In one radar application, the radar
set differentiates between the transmitted and reflected wave to determine the speed of the moving object. 1-26
The Doppler method is the best means of detecting fast-moving objects that do not require range
resolution. As a moving object approaches the transmitter, it encounters and reflects more waves per unit of time.
The amount of frequency shift produced is very small in relation to the carrier frequency. This is because the
velocity of propagation of the signal is very high compared to the speed of the target. However, because the
carrier frequencies used in radar are high, larger frequency shifts (in the audio- frequency range) are produced.
The amount of shift is proportional to the speed of the reflecting object. One-quarter cycle shift at 10,000
megahertz will provide speed measurements accurate to a fraction of a percent. If an object is moving, its
velocity, relative to the radar, can be detected by comparing the transmitter frequency with the echo frequency
(which differs because of the Doppler shift). The Difference or BEAT Frequency, sometimes called the DOPPLER
Frequency (fd), is related to object velocity. The separation of the background and the radar contact is based
on the Doppler frequency that is caused by the reflection of the signal from a moving object. Disadvantages of the
Doppler system are that it does not determine the range of the object, nor is it able to differentiate between
objects when they lie in the same direction and are traveling at the same speed. Moreover, it does not "see"
stationary or slow- moving objects, which a pulse radar system can detect.
To track an object with CW Doppler, you must determine the radar range. Since the Doppler frequency is not
directly related to range, another method is needed to determine object range. By using two separate transmitters
that operate at two different frequencies (f1 and f 2), you can determine range by measuring the relative phase
difference between the two Doppler frequencies. In such a system, a mixer is used to combine the two transmitted
frequencies and to separate the two received frequencies. This permits the use of one transmitting and receiving
antenna. Instead of using two transmitter frequencies, you can find the range by sweeping the transmitter frequency
uniformly in time to cover the frequency range from f1 to f2. The beat, or difference, frequency between the
transmitted and received signals is then a function of range. In this type of radar, the velocity as well as range
is measured.
Q24. The Doppler effect causes a change in what aspect of RF energy that strikes a moving object?
Q25. The Doppler variation is directly proportional to what radar contact characteristic? Q26.
The Doppler method of object detection is best for what type objects? Q27. The beat frequency in a
swept-frequency transmitter provides what contact information? Frequency-MODULATION METHOD
In the frequency-modulation method, the transmitter radiates radio-frequency waves. The frequency of
these RF waves is continually increasing and decreasing from a fixed reference frequency. At any instant, the
frequency of the returned signal differs from the frequency of the radiated signal. The amount of the difference
frequency is determined by the time it took the signal to travel the distance from the transmitter to the object. An example of a frequency-modulated signal, plotted against time, is shown in figure 1-21. As shown, the
420-megahertz frequency increases linearly to 460 megahertz and then quickly drops to 420 megahertz again. When
the frequency drops to 420 megahertz the frequency cycle starts over again. 1-27
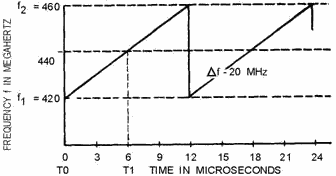
Figure 1-21. - Frequency-modulation chart. The frequency regularly changes 40 megahertz with respect to time; therefore, its value at any time during its
cycle can be used as the basis for computing the time elapsed after the start of the frequency cycle. For example,
at T0 the transmitter sends a 420-megahertz signal toward an object. It strikes the object and returns to the
receiver at T1, when the transmitter is sending out a new frequency of 440 megahertz. At T1, the 420-megahertz
returned signal and the 440-megahertz transmitter signal are fed to the receiver simultaneously. When the two
signals are mixed in the receiver, a beat frequency results. The beat frequency varies directly with the distance
to the object, increasing as the distance increases. Using this information, you can calibrate a device that
measures frequency to indicate range. This system works well when the detected object is stationary. It is used in aircraft altimeters which give a continuous reading of the height above the earth of the aircraft. The
system is not satisfactory for locating moving objects. This is because moving targets produce a frequency shift
in the returned signal because of the Doppler effect; this affects the accuracy of the range measurement.
PULSE-MODULATION METHOD The pulse-modulation method of energy transmission was analyzed to some
extent earlier in this chapter. As the previous discussions indicated, radio-frequency energy can also be
transmitted in very short bursts, called pulses. These pulses are of extremely short time duration, usually on the
order of 0.1 microsecond to approximately 50 microseconds. In this method, the transmitter is turned on for a very
short time and the pulse of radio-frequency energy is transmitted, as shown in view a of figure 1-22. The
transmitter is then turned off, and the pulse travels outward from the transmitter at the velocity of light (view
B). When the pulse strikes an object (view C), it is reflected and begins to travel back toward the radar system,
still moving at the same velocity (view D). The pulse is then received by the radar system (view E). The time
interval between transmission and reception is computed and converted into a visual indication of range in miles
or yards. The radar cycle then starts over again by transmitting another pulse (view F). This method does not
depend on the relative frequency of the returned signal or on the motion of the target; therefore, it has an
important advantage over CW and FM methods. 1-28
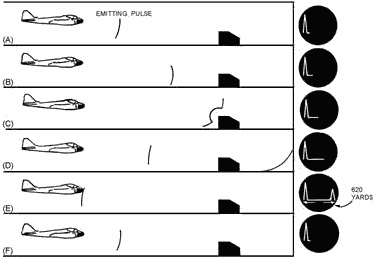
Figure 1-22. - Pulse detection. PULSE-DOPPLER METHOD Pulse radar systems may be modified to use the Doppler effect to
detect a moving object. a requirement for any Doppler radar is COHERENCE; that is, some definite phase
relationship must exist between the transmitted frequency and the reference frequency, which is used to detect the
Doppler shift of the receiver signal. Moving objects are detected by the phase difference between the target
signal and background noise components. Phase detection of this type relies on coherence between the transmitter
frequency and the receiver reference frequency. In coherent detection, a stable CW reference oscillator
signal, which is locked in phase with the transmitter during each transmitted pulse, is mixed with the echo signal
to produce a beat or difference signal. Since the reference oscillator and the transmitter are locked in phase,
the echoes are effectively compared with the transmitter in frequency and phase. The phase relationships
of the echoes from fixed objects to the transmitter is constant and the amplitude of the beat signal remains
constant. a beat signal of varying amplitude indicates a moving object. This is because the phase difference
between the reference oscillator signal and the echo signal changes as the range to the reflecting object changes.
The constant amplitude beat signal is filtered out in the receiver. The beat signal of varying amplitude is sent
to the radar indicator scope for display.
Q28. What factor determines the difference between the transmitted frequency and the received frequency in an
FM transmitter? Q29. What type of objects are most easily detected by an FM system?
Q30. What transmission method does NOT depend on relative frequency or target motion? 1-29
Q31. What transmission method uses a stable CW reference oscillator, which is locked in phase with the
transmitter frequency? Radar Classification and use Radar systems, like cars, come in a variety of sizes and have different performance specifications. Some radar
systems are used for air-traffic control at airports and others are used for long-range surveillance and
early-warning systems. a radar system is the heart of a missile guidance system. Small portable radar systems that
can be maintained and operated by one person are available as well as systems that occupy several large rooms.
MILITARY Classification of Radar Systems The large number of radar systems used by the military has
forced the develoPMent of a joint- services classification system for accurate identification. The Federal
Aviation Agency (FAA) also makes extensive use of radar systems for commercial aircraft in-flight and landing
control, but does not use the military classification system. Radar systems are usually classified
according to specific function and installation vehicle. Some common examples are listed below: 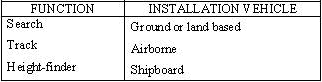
The joint-service standardized classification system further divides these broad categories for more precise
identification. Table 1-1 is a listing of equipment identification indicators. use of the table to identify a
particular radar system is illustrated in figure 1-23. Note that for simplicity, only a portion of the table has
been used in the illustration.
1-30
- |
Matter, Energy,
and Direct Current |
- |
Alternating Current and Transformers |
- |
Circuit Protection, Control, and Measurement |
- |
Electrical Conductors, Wiring Techniques,
and Schematic Reading |
- |
Generators and Motors |
- |
Electronic Emission, Tubes, and Power Supplies |
- |
Solid-State Devices and Power Supplies |
- |
Amplifiers |
- |
Wave-Generation and Wave-Shaping Circuits |
- |
Wave Propagation, Transmission Lines, and
Antennas |
- |
Microwave Principles |
- |
Modulation Principles |
- |
Introduction to Number Systems and Logic Circuits |
- |
- Introduction to Microelectronics |
- |
Principles of Synchros, Servos, and Gyros |
- |
Introduction to Test Equipment |
- |
Radio-Frequency Communications Principles |
- |
Radar Principles |
- |
The Technician's Handbook, Master Glossary |
- |
Test Methods and Practices |
- |
Introduction to Digital Computers |
- |
Magnetic Recording |
- |
Introduction to Fiber Optics |
Note: Navy Electricity and Electronics Training
Series (NEETS) content is U.S. Navy property in the public domain. |
|