Module 18 - Radar PrinciplesPages
i,
1-1, 1-11,
1-21,
1-31,
1-41,
2-1,
2-11,
2-21,
2-31,
2-41,
3-1,
3-11,
3-21,
4-1,
4-11,
4-21,
AI-1,
AII-1,
Index-1 to 3
Noise
The word NOIsE is a carryover from sound-communications equipment terminology. Noise voltages in
sound equipment produce actual noise in the loudspeaker output. In radar, noise voltages result in erratic, random
deflection or intensity of the indicator sweep that can mask small return signals. Were it not for noise,
the maximum range at which an object would be detectable by radar could be extended almost infinitely. Objects at
great range return exceedingly small echoes. However, without noise, almost any signal could be amplified to a
usable level if enough stages were added to the receiver. Because of noise, the signal detection limit or
sensitivity level of a receiver is reached when the signal level falls below the noise level to such an extent as
to be obscured. a simple increase of amplification is of no help because both signal and noise are amplified at
the same rate. In the radar portion of the RF spectrum, external sources of noise interference are usually
negligible; consequently, the sensitivity that can be achieved in a radar receiver is usually determined by the
noise produced in the receiver. Not only must noise be kept down, but everything possible must be done to minimize
attenuation of the video signal (echo) before it is amplified. Gain The Gain of a
radar receiver must be very high. This is because the strength of the signal at the antenna is at a level of
microvolts and the required output to the indicator is several volts. The gain of a radar receiver is roughly in
the range of 106 to 10 8. Feedback, or REGENERATION, is one of the most serious difficulties in the design of an
amplifier with such high gain. Special precautions must be taken to avoid feedback. Such precautions include
careful shielding, decoupling (isolation) between voltage supplies for the different tubes, and amplification at
different frequencies in separate groups of stages.
Tuning The radar receiver requires a limited tuning range to compensate for transmitter and local
oscillator frequency changes because of variations in temperature and loading. Microwave radar receivers usually use automatic frequency control (afc) for this purpose. Distortion If distortion
occurs in the receiver, the time interval between the transmitted pulse and the received pulse changes, thereby
affecting range accuracy.
Blocking BlockING refers to a condition of the receiver in which the voltage pulse at
the receiver input is too large. As a result, for a short time after the pulse, the receiver is insensitive or
blocked to signals below a certain level. This condition results from one or more of the amplifier stages in
the receiver being overdriven. After a strong pulse, the receiver may be biased to a point at which it will not
amplify small signals. Recovery after blocking may be only a fraction of a microsecond, or it may take several
hundred microseconds, depending upon the point in the receiver at which blocking occurs. To detect a weak echo
immediately following a strong one, the receiver must have a short BlockING RECOVery TIME. The blocking
itself must be minimized as much as possible. If a portion of the transmitted pulse leaks into the receiver input,
then the receiver may be blocked and not show small, nearby objects. In most receivers, blocking is minimized from
this cause by a duplexer. The duplexer protects the receiver by isolating it during the transmitted pulse. 2-31
RECEIVER Block Diagram The SUPERHETERODYNE receiver is almost always used in
microwave radar systems. a typical superheterodyne radar receiver is shown in figure 2-23. a receiver of this type
meets all the requirements listed above. Signals from the antenna enter the receiver via the duplexer. a low-noise
RF amplifier is usually the first stage of modern radar receivers. Some receivers, however, send the antenna
signal directly to the mixer, as shown by the dashed path. The low-noise amplifiers used in modern systems are
usually solid-state devices, such as tunnel-diode, parametric, or microwave transistor amplifiers. 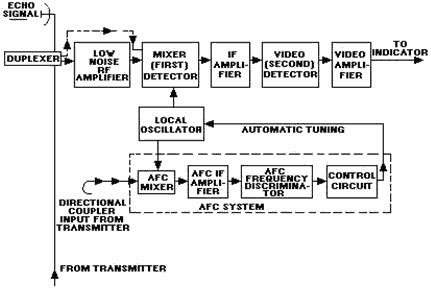
Figure 2-23. - Typical superheterodyne radar receiver. The MIXER stage is often called the First DETECTOR. The function of this stage is to convert the
received RF energy to a lower, intermediate frequency (IF) that is easier to amplify and manipulate
electronically. The intermediate frequency is usually 30 or 60 megahertz. It is obtained by heterodyning the
received signal with a local-oscillator signal in the mixer stage. The mixer stage converts the received signal to
the lower IF signal without distorting the data on the received signal. After conversion to the
intermediate frequency, the signal is amplified in several IF Amplifier stages. Most of the gain of the receiver
is developed in the IF amplifier stages. The overall bandwidth of the receiver is often determined by the
bandwidth of the IF stages. The output of the IF amplifiers is applied to the SECOND DETECTOR. It is then
rectified and passed through one or more stages of amplification in the video amplifier(s). The output stage of
the receiver is normally an emitter follower. The low-impedance output of the emitter follower matches the
impedance of the cable. The video pulses are coupled through the cable to the indicator for video display on the
crt. 2-32
As in all superheterodyne receivers, controlling the frequency of the local oscillator keeps the
receiver tuned. Since this tuning is critical, some form of automatic frequency control (afc) is essential to
avoid constant manual tuning. Automatic frequency control circuits mix an attenuated portion of the transmitted
signal with the local oscillator signal to form an IF signal. This signal is applied to a frequency-sensitive
discriminator that produces an output voltage proportional in amplitude and polarity to any change in IF
frequency. If the IF signal is at the discriminator center frequency, no discriminator output occurs. The center
frequency of the discriminator is essentially a reference frequency for the IF signal. The output of the
DIsCRIMINATOR provides a control voltage to maintain the local oscillator at the correct frequency. Different receiving systems may vary in the type of coupling between stages, the type of mixer, the detector, the
local oscillator, and the number of stages of amplification at the different frequencies. However, the receiver is
always designed to have as little noise as possible. It is also designed to have sufficient gain so that noise,
rather than lack of gain, limits the smallest visible signal. RECEIVER COMPONENTS This section will analyze in more detail the operation of the
receiver circuits mentioned above. The circuits discussed are usually found in some form in all radar
superheterodyne receivers.
Low-Noise Amplifier Low-NOIsE AmplifierS, sometimes called PREAMPS, are found in most
modern radar receivers. As previously mentioned, these amplifiers are usually solid-state microwave amplifiers.
The most common types are tunnel diode and parametric amplifiers. These amplifiers are discussed in detail in
NEETS, Module 11, Microwave Principles. Some older systems may still use a traveling-wave tube (twt) as a
low-noise first stage amplifier. However, the solid-state amplifiers produce lower noise levels and more gain.
Local Oscillator
Most radar receivers use a 30 or 60 megahertz intermediate frequency. The IF is produced by mixing a
local oscillator signal with the incoming signal. The local oscillator is, therefore, essential to efficient
operation and must be both tunable and very stable. For example, if the local oscillator frequency is 3,000
megahertz, a frequency change of 0.1 percent will produce a frequency shift of 3 megahertz. This is equal to the
bandwidth of most receivers and would greatly decrease receiver gain. The power output requirement for
most local oscillators is small (20 to 50 milliwatts) because most receivers use crystal mixers that require very
little power. The local oscillator output frequency must be tunable over a range of several megahertz in
the 4,000- megahertz region. The local oscillator must compensate for any changes in the transmitted frequency and
maintain a constant 30 or 60 megahertz difference between the oscillator and the transmitter frequency. a local
oscillator that can be tuned by varying the applied voltage is most desirable. The REFLEX KLYSTRON is
often used as a local oscillator because it meets all the requirements mentioned above. The reflex klystron is a
very stable microwave oscillator that can be tuned by changing the repeller voltage. Most radar systems use an automatic frequency control (afc) circuit to control the output of the local oscillator. a block diagram of
a typical afc circuit is included in figure 2-23. Note that the afc circuits form a closed loop. This circuit is,
in fact, often called the afc loop. 2-33
A sample of the transmitter energy is fed through the afc mixer and an IF amplifier to a
discriminator. The output of the discriminator is a dc error voltage that indicates the degree of mistuning
between the transmitter and the local oscillator. In this particular example let's assume that the IF is 30
megahertz. If the output of the mixer is correct, the discriminator will have no output. If the mixer output is
above 30 megahertz, the output of the discriminator will be positive dc pulses; if the mixer output is below 30
megahertz, the discriminator output will be negative dc pulses. In either case, this output is fed through an
amplifier to the control circuit. The control circuit adjusts the operating frequency of the local oscillator so
that no mistuning exists and the IF is 30 megahertz. In this example the local oscillator is a reflex klystron and
the control circuit provides he repeller plate voltage for the klystron; thus, the klystron directly controls the
local oscillator frequency. In this manner the local oscillator is maintained exactly 30 megahertz below the
transmitter frequency. Q34. What is the greatest limiting factor in a receiver's detectable range?
Q35. What type of receiver is most often used in radar systems? Q36. What IF frequencies are normally used in radar receivers? Q37. Which component of the receiver
produces the signal that is mixed with the received signal to produce the IF signal? Mixer
Many older radar receivers do not use a low-noise amplifier as the receiver front end; they simply
send the echo signal directly to a crystal mixer stage. a crystal is used rather than an electron-tube diode
because, at microwave frequencies, the tube would generate excessive noise. Electron tubes are also limited by the
effects of transit time at microwave frequencies. The crystal most commonly used is the point-contact crystal
diode; however, recent develoPMents in the field of solid-state microwave devices may soon replace the
point-contact diode with devices that produce even less noise. The Schottky-barrier diode is an example of a
relatively recent develoPMent that produces less noise than the point-contact crystal. The simplest type
of radar mixer is the Single ENDED or UNBALANCED Crystal MIXER, shown in figure 2-24. The mixer illustrated uses a
tuned section of coaxial transmission line one-half wavelength long. This section matches the crystal to the
signal echo and the local oscillator inputs. Local oscillator injection is accomplished by means of a probe. In
the coaxial assembly, the signal is injected by means of a slot. This slot would normally be inserted in the
duplexer waveguide assembly and be properly oriented to provide coupling of the returned signal. In this
application, the unwanted signals at the output of the mixer (carrier frequency, the local oscillator frequency,
and sum of these two signals) are effectively eliminated by a resonant circuit tuned to the intermediate, or
difference frequency. One advantage of the unbalanced crystal mixer is its simplicity. It has one major
disadvantage; its inability to cancel local oscillator noise. Difficulty in detecting weak signals will exist if
noise is allowed to pass through the mixer along with the signal. 2-34
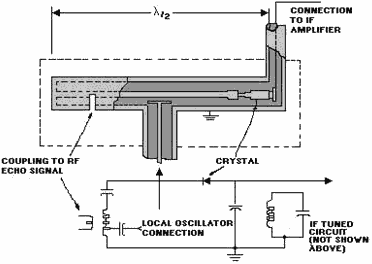
Figure 2-24. - Single-ended crystal mixer. One type of mixer which cancels local oscillator noise is the BALANCED, OR HYBRID, MIXER (sometimes
called the MAGIC T). Figure 2-25 shows this type of mixer. In hybrid mixers, crystals are inserted directly into
the waveguide. The crystals are located one-quarter wavelength from their respective short-circuited waveguide
ends (a point of maximum voltage along a tuned line). The crystals are also connected to a balanced transformer,
the secondary of which is tuned to the desired IF. The local oscillator signal is introduced into the waveguide
local oscillator arm and distributes itself as shown in view a of figure 2-26. Observe that the local oscillator
signal is in phase across the crystals. In view B the echo signal is introduced into the echo signal arm of the
waveguide and is out of phase across the crystals. The resulting fields are shown in view C. 2-35
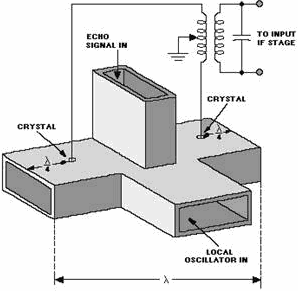
Figure 2-25. - Balanced (hybrid) crystal mixer. 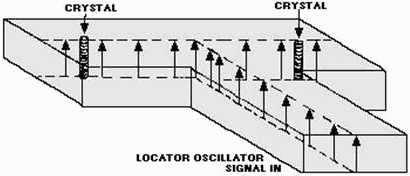
Figure 2-26A. - Balanced mixer fields. WAVEGUIDE and LOCAL Oscillator ARM. 2-36
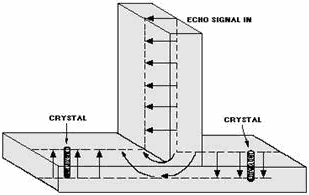
Figure 2-26B. - Balanced mixer fields. WAVEGUIDE and ECHO Signal ARM. 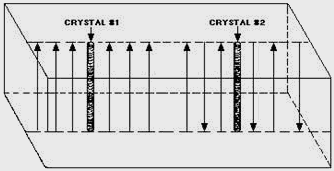
Figure 2-26C. - Balanced mixer fields. WAVEGUIDE. A difference in phase exists between echo signals applied across the two crystals. The signal applied to
the crystals from the local oscillator is in phase. Therefore, at some point both signals applied to crystal #1
will be in phase, and the signals applied to crystal #2 will be out of phase. This means that an IF signal of one
polarity will be produced across crystal #1 and an IF signal of the opposite polarity will be produced across
crystal #2. When these two signals are applied to the balanced output transformer (figure 2-25), they will add.
Outputs of the same polarity will cancel across the balanced transformer. This action eliminates the noise of the
local oscillator. Noise components introduced from the local oscillator are in phase across the crystals and are,
therefore, cancelled in the balanced transformer. The RF characteristics of the crystals must be nearly equal, or
the noise of the local oscillator will not completely cancel. Note that only the noise produced by the local
oscillator is canceled. Noise arriving with the echo signal is not affected. 2-37
IF Amplifier Stage The IF Amplifier SECTION of a radar receiver determines the
gain, signal-to-noise ratio, and effective bandwidth of the receiver. The typical IF amplifier (commonly called an
IF strip) usually contains from three to ten amplifier stages. The IF amplifier has the capability to vary both
the bandpass and the gain of a receiver. Normally, the bandpass is as narrow as possible without affecting the
actual signal energy. When a selection of pulse widths is available, such as short and long pulses, the bandpass
must be able to match the bandwidth of the two different signals. Gain must be variable to provide a constant
voltage output for input signals of different amplitudes. Figure 2-27 is a block diagram of an IF amplifier that
meets these requirements. 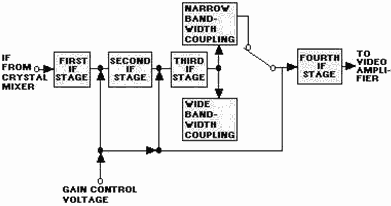
Figure 2-27. - IF amplifier block diagram. The most critical stage of the IF section is the input (first stage). The quality of this stage
determines the noise figure of the receiver and the performance of the entire receiving system with respect to
detection of small objects at long ranges. Gain and bandwidth are not the only considerations in the design of the
first IF stage. a consideration perhaps of more importance is noise generation. Noise generation in this stage
must be low. Noise generated in the input IF stage will be amplified by succeeding stages and may exceed the echo
signal in strength. Detectors The detector in a microwave receiver serves to convert the IF pulses into video
pulses. After amplification, these are applied to the indicator. The simplest form of detector, and the one most
commonly used in microwave receivers, is the DIODE DETECTOR. A diode detector circuit is shown in view a
of figure 2-28. The secondary of T1 and C1 form a tuned circuit that is resonant at the intermediate frequency.
Should an echo pulse of sufficient amplitude be received, the voltage (ei) developed across the tuned circuit is
an IF pulse. Its shape is indicated by the dashed line in view B. Positive excursions of e i cause no current to
flow through the diode. However, negative excursions result in a flow of diode current and a subsequent negative
voltage (eo) to be developed across R1 and C2. Between peak negative voltage excursions of the ei wave, capacitor
C2 discharges through R1. Thus, the eo waveform is a negative video pulse with sloping edges and 2-38
superimposed IF ripple, as indicated by the solid line in view B. a negative polarity of the output
pulse is ordinarily preferred, but a positive pulse may be obtained by reversing the connections of the diode. In
view A, inductance L1, in combination with wiring capacitance and C2, forms a low-pass filter. This filter
attenuates the IF components in the eo waveform but results in a minimum loss of video high-frequency components. 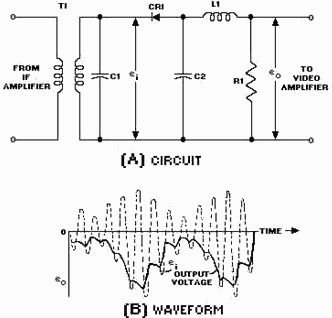
Figure 2-28. - Diode detector. Video Amplifiers The video amplifier receives pulses from the detector and
amplifies these pulses for application to the indicating device. a video amplifier is fundamentally an RC coupled
amplifier that uses high-gain transistors or pentodes. However, a video amplifier must be capable of a relatively
wide frequency response. Stray and interelectrode capacitances reduce the high-frequency response of an amplifier,
and the reactance of the coupling capacitor diminishes the low-frequency response. These problems are overcome by
the use of Frequency Compensation NetworkS in the video amplifier. The types of frequency compensation networks
that may be used in a video amplifier stage are discussed in detail in NEETS, Module 8, Introduction to
Amplifiers. Q38. What receiver circuit actually produces the IF frequency? Q39. The IF amplifiers
are connected in what amplifier configuration?
Q40. Which receiver component converts the IF pulses to video pulses? 2-39
RECEIVER Special Circuits The performance efficiency of radar receivers is often
greatly decreased by interference from one or more of several possible sources. Weather and sea return are the
most common of these interference sources, especially for radar systems that operate above 3,000 megahertz.
Unfavorable weather conditions can completely mask all radar returns and render the system useless.
Electromagnetic interference from external sources, such as the deliberate interference by an enemy, called
jamming or electronic counter measures (ECM), can also render a radar system useless. Many special circuits have
been designed to help the radar receiver counteract the effects of external interference. These circuits are
called Video ENHANCEMENT FEATURES, ANTIJAMMING Circuits, or ELECTRONIC COUNTER-COUNTERMEASURES (ECCM) Circuits.
This section will discuss, in general terms, some of the more common video enhancement features associated with
radar receivers. Automatic Gain Control (AGC) Most radar receivers use some means to
control the overall gain. This usually involves the gain of one or more IF amplifier stages. Manual gain control
by the operator is the simplest method. Usually, some more complex form of automatic gain control (agc) or
instantaneous automatic gain control (iagc) is used during normal operation. Gain control is necessary to adjust
the receiver sensitivity for the best reception of signals of widely varying amplitudes. Agc and iagc circuits are
designed with, a shut-off feature so that receiver gain may be adjusted manually. In this way, manual gain control
can be used to adjust for best reception of a particular signal. The simplest type of agc adjusts the IF amplifier bias (and gain) according to the average level of the
received signal. Agc is not used as frequently as other types of gain control because of the widely varying
amplitudes of radar return signals. With agc, gain is controlled by the largest received signals. When
several radar signals are being received simultaneously, the weakest signal may be of greatest interest. Iagc is used more frequently because it adjusts receiver gain for each signal. The iagc circuit is essentially a
wide-band, dc amplifier. It instantaneously controls the gain of the IF amplifier as the radar return signal
changes in amplitude. The effect of iagc is to allow full amplification of weak signals and to decrease the
amplification of strong signals. The range of iagc is limited, however, by the number of IF stages in which gain
is controlled. When only one IF stage is controlled, the range of iagc is limited to approximately 20 dB. When
more than one IF stage is controlled, iagc range can be increased to approximately 40 dB.
Sensitivity Time Control (STC) In radar receivers, the wide variation in return signal amplitudes
make adjustment of the gain difficult. The adjustment of receiver gain for best visibility of nearby target return
signals is not the best adjustment for distant target return signals. Circuits used to adjust amplifier gain with
time, during a single pulse-repetition period, are called stc circuits. Sensitivity time-control circuits
apply a bias voltage that varies with time to the IF amplifiers to control receiver gain. Figure 2-29 shows a
typical stc waveform in relation to the transmitted pulse. When the transmitter fires, the stc circuit decreases
the receiver gain to zero to prevent the amplification of any leakage energy from the transmitted pulse. At the
end of the transmitted pulse, the stc voltage begins to rise, gradually increasing the receiver gain to maximum.
The stc voltage effect on receiver gain is usually limited to approximately 50 miles. This is because close-in
targets are most likely to saturate the receiver; beyond 50 miles, stc has no affect and the receiver operates
normally.
2-40
- |
Matter, Energy,
and Direct Current |
- |
Alternating Current and Transformers |
- |
Circuit Protection, Control, and Measurement |
- |
Electrical Conductors, Wiring Techniques,
and Schematic Reading |
- |
Generators and Motors |
- |
Electronic Emission, Tubes, and Power Supplies |
- |
Solid-State Devices and Power Supplies |
- |
Amplifiers |
- |
Wave-Generation and Wave-Shaping Circuits |
- |
Wave Propagation, Transmission Lines, and
Antennas |
- |
Microwave Principles |
- |
Modulation Principles |
- |
Introduction to Number Systems and Logic Circuits |
- |
- Introduction to Microelectronics |
- |
Principles of Synchros, Servos, and Gyros |
- |
Introduction to Test Equipment |
- |
Radio-Frequency Communications Principles |
- |
Radar Principles |
- |
The Technician's Handbook, Master Glossary |
- |
Test Methods and Practices |
- |
Introduction to Digital Computers |
- |
Magnetic Recording |
- |
Introduction to Fiber Optics |
Note: Navy Electricity and Electronics Training
Series (NEETS) content is U.S. Navy property in the public domain. |
|