Module 10 - Introduction to Wave Propagation, Transmission Lines, and Antennas
Pages i,
1-1,
1-11,
1-21,
1-31,
1-41,
2-1,
2-11,
2-21,
2-31,
2-40,
3-1,
3-11,
3-21,
3-31,
3-41,
3-51,
4-1,
4-11,
4-21,
4-31,
4-41,
4-51, Index
Chapter 3
Principles of Transmission Lines
Learning Objectives
Upon completion of this chapter, you will be able to:
1. State what a transmission line is and how transmission lines are
used.
2. Explain the operating principles of transmission lines.
3. Describe the five types of transmission lines.
4. State the length of a transmission line.
5. Explain the theory of the transmission line.
6. Define the term LUMPED CONSTANTS in relation to a transmission
line.
7. Define the term Distributed CONSTANTS in relation to a transmission
line.
8. Define LEAKAGE CURRENT.
9. Describe how the electromagnetic lines of force around a transmission
line are affected by the distributed constants.
10. Define the term Characteristic Impedance and explain how it affects
the transfer of energy along a transmission line.
11. State how the energy transfer along a transmission line is affected
by characteristic impedance and the infinite line.
12. Identify the cause of and describe the characteristics of reflections
on a transmission line.
13. Define the term Standing WAVES as applied to a transmission line.
14. Describe how standing waves are produced on a transmission line
and identify the types of terminations.
15. Describe the types of standing-wave ratios.
Introduction to Transmission Lines
A Transmission LINE is a device designed to guide electrical energy from one
point to another. It is used, for example, to transfer the output RF energy of a
transmitter to an antenna. This energy will not travel through normal electrical
wire without great losses. Although the antenna can be connected directly to the
transmitter, the antenna is usually located some distance away from the transmitter.
On board ship,
3-1
the transmitter is located inside a radio room and its associated antenna is
mounted on a mast. a transmission line is used to connect the transmitter and the
antenna.
The transmission line has a single purpose for both the transmitter and the antenna.
This purpose is to transfer the energy output of the transmitter to the antenna
with the least possible power loss. How well this is done depends on the special
physical and electrical characteristics (impedance and resistance) of the transmission
line.
Terminology
All transmission lines have two ends (see figure 3-1). The end of a two-wire
transmission line connected to a source is ordinarily called the Input END or the
GENERATOR END. Other names given to this end are Transmitter END, SENDING END, and
SOURCE. The other end of the line is called the Output END or RECEIVING END. Other
names given to the output end are Load END and SINK.

Figure 3-1. - Basic transmission line.
You can describe a transmission line in terms of its impedance. The ratio of
voltage to current (Ein/Iin) at the input end is known as the Input Impedance (Zin).
This is the impedance presented to the transmitter by the transmission line and
its load, the antenna. The ratio of voltage to current at the output (Eout/Iout)
end is known as the Output Impedance (Zout). This is the impedance presented to
the load by the transmission line and its source. If an infinitely long transmission
line could be used, the ratio of voltage to current at any point on that transmission
line would be some particular value of impedance. This impedance is known as the
Characteristic Impedance.
Q1. What connecting link is used to transfer energy from a radio
transmitter to its antenna located on the mast of a ship?
Q2. What term is used for the end of the transmission line that is
connected to a transmitter?
Q3. What term is used for the end of the transmission line that is
connected to an antenna?
TYPES of Transmission MEDIUMS
The Navy uses many different types of Transmission MEDIUMS in its electronic
applications. Each medium (line or wave guide) has a certain characteristic impedance
value, current-carrying capacity, and physical shape and is designed to meet a particular
requirement.
3-2
The five types of transmission mediums that we will discuss in this chapter include
PARALLEL-LINE, TWIsTED PAIR, SHIELDED PAIR, COAXIAL LINE, and WAVEGUIDES. The use
of a particular line depends, among other things, on the applied frequency, the
power-handling capabilities, and the type of installation.
Note: In the following paragraphs, we will mention LOSSES several
times. We will discuss these losses more thoroughly under "LOSSES IN Transmission
Lines."
Two-Wire Open Line
One type of parallel line is the TWO-Wire OPEN LINE illustrated in figure 3-2.
This line consists of two wires that are generally spaced from 2 to 6 inches apart
by insulating spacers. This type of line is most often used for power lines, rural
telephone lines, and telegraph lines. It is sometimes used as a transmission line
between a transmitter and an antenna or between an antenna and a receiver. An advantage
of this type of line is its simple construction. The principal disadvantages of
this type of line are the high radiation losses and electrical noise pickup because
of the lack of shielding. Radiation losses are produced by the changing fields created
by the changing current in each conductor.
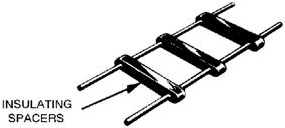
Figure 3-2. - Parallel two-wire line.
Another type of parallel line is the TWO-Wire RIBBON (TWIN LEAD) illustrated
in figure 3-3. This type of transmission line is commonly used to connect a television
receiving antenna to a home television set. This line is essentially the same as
the two-wire open line except that uniform spacing is assured by embedding the two
wires in a low-loss dielectric, usually polyethylene. Since the wires are embedded
in the thin ribbon of polyethylene, the dielectric space is partly air and partly
polyethylene.

Figure 3-3. - Two-wire ribbon type line.
3-3
Twisted Pair
The TWIsTED PAIR transmission line is illustrated in figure 3-4. As the name
implies, the line consists of two insulated wires twisted together to form a flexible
line without the use of spacers. It is not used for transmitting high frequency
because of the high dielectric losses that occur in the rubber insulation. When
the line is wet, the losses increase greatly.

Figure 3-4. - Twisted pair.
Shielded Pair
The SHIELDED PAIR, shown in figure 3-5, consists of parallel conductors separated
from each other and surrounded by a solid dielectric. The conductors are contained
within a braided copper tubing that acts as an electrical shield. The assembly is
covered with a rubber or flexible composition coating that protects the line from
moisture and mechanical damage. Outwardly, it looks much like the power cord of
a washing machine or refrigerator.

Figure 3-5. - Shielded pair.
The principal advantage of the shielded pair is that the conductors are balanced
to ground; that is, the capacitance between the wires is uniform throughout the
length of the line. This balance is due to the uniform spacing of the grounded shield
that surrounds the wires along their entire length. The braided copper shield isolates
the conductors from stray magnetic fields.
Coaxial Lines
There are two types of COAXIAL Lines, RIGID (AIR) COAXIAL LINE and FLEXIBLE (SOLID)
COAXIAL LINE. The physical construction of both types is basically the same; that
is, each contains two concentric conductors.
3-4
The rigid coaxial line consists of a central, insulated wire (inner conductor)
mounted inside a tubular outer conductor. This line is shown in figure 3-6. In some
applications, the inner conductor is also tubular. The inner conductor is insulated
from the outer conductor by insulating spacers or beads at regular intervals. The
spacers are made of Pyrex, polystyrene, or some other material that has good insulating
characteristics and low dielectric losses at high frequencies.

Figure 3-6. - Air coaxial line.
The chief advantage of the rigid line is its ability to minimize radiation losses.
The electric and magnetic fields in a two-wire parallel line extend into space for
relatively great distances and radiation losses occur. However, in a coaxial line
no electric or magnetic fields extend outside of the outer conductor. The fields
are confined to the space between the two conductors, resulting in a perfectly shielded
coaxial line. Another advantage is that interference from other lines is reduced.
The rigid line has the following disadvantages: (1) it is expensive to construct;
(2) it must be kept dry to prevent excessive leakage between the two conductors;
and (3) although high-frequency losses are somewhat less than in previously mentioned
lines, they are still excessive enough to limit the practical length of the line.
Leakage caused by the condensation of moisture is prevented in some rigid line
applications by the use of an inert gas, such as nitrogen, helium, or argon. It
is pumped into the dielectric space of the line at a pressure that can vary from
3 to 35 pounds per square inch. The inert gas is used to dry the line when it is
first installed and pressure is maintained to ensure that no moisture enters the
line.
Flexible coaxial lines (figure 3-7) are made with an inner conductor that consists
of flexible wire insulated from the outer conductor by a solid, continuous insulating
material. The outer conductor is made of metal braid, which gives the line flexibility.
Early attempts at gaining flexibility involved using rubber insulators between the
two conductors. However, the rubber insulators caused excessive losses at high frequencies.
3-5

Figure 3-7. - Flexible coaxial line.
Because of the high-frequency losses associated with rubber insulators, polyethylene
plastic was developed to replace rubber and eliminate these losses. Polyethylene
plastic is a solid substance that remains flexible over a wide range of temperatures.
It is unaffected by seawater, gasoline, oil, and most other liquids that may be
found aboard ship. The use of polyethylene as an insulator results in greater high-frequency
losses than the use of air as an insulator. However, these losses are still lower
than the losses associated with most other solid dielectric materials.
Waveguides
The WAVEGUIDE is classified as a transmission line. However, the method by which
it transmits energy down its length differs from the conventional methods. Waveguides
are cylindrical, elliptical, or rectangular (cylindrical and rectangular shapes
are shown in figure 3-8). The rectangular waveguide is used more frequently than
the cylindrical waveguide.

Figure 3-8. - Waveguides.
The term waveguide can be applied to all types of transmission lines in the sense
that they are all used to guide energy from one point to another. However, usage
has generally limited the term to mean a hollow metal tube or a dielectric transmission
line. In this chapter, we use the term waveguide only to mean "hollow metal tube."
It is interesting to note that the transmission of electromagnetic energy along
a waveguide travels at a velocity somewhat slower than electromagnetic energy traveling
through free space.
A waveguide may be classified according to its cross section (rectangular, elliptical,
or circular), or according to the material used in its construction (metallic or
dielectric). Dielectric waveguides are
3-6
seldom used because the dielectric losses for all known dielectric materials
are too great to transfer the electric and magnetic fields efficiently.
The installation of a complete waveguide transmission system is somewhat more
difficult than the installation of other types of transmission lines. The radius
of bends in the waveguide must measure greater than two wavelengths at the operating
frequency of the equipment to avoid excessive attenuation. The cross section must
remain uniform around the bend. These requirements hamper installation in confined
spaces. If the waveguide is dented, or if solder is permitted to run inside the
joints, the attenuation of the line is greatly increased. Dents and obstructions
in the waveguide also reduce its breakdown voltage, thus limiting the waveguide's
power-handling capability because of possible arc over. Great care must be exercised
during installation; one or two carelessly made joints can seriously inhibit the
advantage of using the waveguide.
We will not consider the waveguide operation in this module, since waveguide
theory is discussed in NEETS, Module 11, Microwave Principles.
Q4. List the five types of transmission lines in use today.
Q5. Name two of the three described uses of a two-wire open line.
Q6. What are the two primary disadvantages of a two-wire open line?
Q7. What type of transmission line is often used to connect a television
set to its antenna?
Q8. What is the primary advantage of the shielded pair?
Q9. What are the two types of coaxial lines in use today?
Q10. What is the chief advantage of the air coaxial line?
Q11. List the three disadvantages of the air coaxial line.
Q12. List the two common types of waveguides in use today.
LOSSES IN Transmission Lines
The discussion of transmission lines so far has not directly addressed LINE LOSSES;
actually some line losses occur in all lines. Line losses may be any of three types
- COPPER, Dielectric, and Radiation or INDUCTION LOSSES.
Note: Transmission lines are sometimes referred to as RF lines.
In this text the terms are used interchangeably.
Copper Losses
One type of copper loss is I2R LOSS. In RF lines the resistance of the conductors
is never equal to zero. Whenever current flows through one of these conductors,
some energy is dissipated in the form of heat. This heat loss is a Power LOSS. With
copper braid, which has a resistance higher than solid tubing, this power loss is
higher.
Another type of copper loss is due to SKIN EFFECT. When dc flows through a conductor,
the movement of electrons through the conductor's cross section is uniform. The
situation is somewhat different when ac is applied. The expanding and collapsing
fields about each electron encircle other electrons. This phenomenon, called SELF
INDUCTION, retards the movement of the encircled electrons.
3-7
The flux density at the center is so great that electron movement at this point
is reduced. As frequency is increased, the opposition to the flow of current in
the center of the wire increases. Current in the center of the wire becomes smaller
and most of the electron flow is on the wire surface. When the frequency applied
is 100 megahertz or higher, the electron movement in the center is so small that
the center of the wire could be removed without any noticeable effect on current.
You should be able to see that the effective cross-sectional area decreases as the
frequency increases. Since resistance is inversely proportional to the cross-sectional
area, the resistance will increase as the frequency is increased. Also, since power
loss increases as resistance increases, power losses increase with an increase in
frequency because of skin effect.
Copper losses can be minimized and conductivity increased in an RF line by plating
the line with silver. Since silver is a better conductor than copper, most of the
current will flow through the silver layer. The tubing then serves primarily as
a mechanical support.
Dielectric Losses
Dielectric LOSSES result from the heating effect on the dielectric material between
the conductors. Power from the source is used in heating the dielectric. The heat
produced is dissipated into the surrounding medium. When there is no potential difference
between two conductors, the atoms in the dielectric material between them are normal
and the orbits of the electrons are circular. When there is a potential difference
between two conductors, the orbits of the electrons change. The excessive negative
charge on one conductor repels electrons on the dielectric toward the positive conductor
and thus distorts the orbits of the electrons. a change in the path of electrons
requires more energy, introducing a power loss.
The atomic structure of rubber is more difficult to distort than the structure
of some other dielectric materials. The atoms of materials, such as polyethylene,
distort easily. Therefore, polyethylene is often used as a dielectric because less
power is consumed when its electron orbits are distorted.
Radiation and Induction Losses
Radiation and INDUCTION LOSSES are similar in that both are caused by the fields
surrounding the conductors. Induction losses occur when the electromagnetic field
about a conductor cuts through any nearby metallic object and a current is induced
in that object. As a result, power is dissipated in the object and is lost.
Radiation losses occur because some magnetic lines of force about a conductor
do not return to the conductor when the cycle alternates. These lines of force are
projected into space as radiation and this results in power losses. That is, power
is supplied by the source, but is not available to the load.
Q13. What are the three types of line losses associated with transmission
lines?
Q14. Losses caused by skin effect and the I2R (power) loss
are classified as what type of loss?
Q15. What types of losses cause the dielectric material between the
conductors to be heated?
LENGTH of a Transmission LINE
A transmission line is considered to be electrically short when its physical
length is short compared to a quarter-wavelength (1/4λ) of the energy
it is to carry.
Note: In this module, for ease of reading, the value of the
wavelength will be spelled out in some cases, and in other cases, the numerical
value will be used.
3-8
A transmission line is electrically long when its physical length is long compared
to a quarter- wavelength of the energy it is to carry. You must understand that
the terms "short" and "long" are relative ones. For example, a line that has a physical
length of 3 meters (approximately 10 feet) is considered quite short electrically
if it transmits a radio frequency of 30 kilohertz. On the other hand, the same transmission
line is considered electrically long if it transmits a frequency of 30,000 megahertz.
To show the difference in physical and electrical lengths of the lines mentioned
above, compute the wavelength of the two frequencies, taking the 30-kilohertz example
first:

Now, computing the wavelength for the line carrying 30,000 megahertz:

Thus, you can see that a 3-meter line is electrically very short for a frequency
of 30 kilohertz. Also, the 3-meter line is electrically very long for a frequency
of 30,000 megahertz.
When power is applied to a very short transmission line, practically all of it
reaches the load at the output end of the line. This very short transmission line
is usually considered to have practically no electrical properties of its own, except
for a small amount of resistance.
3-9
However, the picture changes considerably when a long line is used. Since most
transmission lines are electrically long (because of the distance from transmitter
to antenna), the properties of such lines must be considered. Frequently, the voltage
necessary to drive a current through a long line is considerably greater than the
amount that can be accounted for by the impedance of the load in series with the
resistance of the line.
Transmission LINE THEORY
The electrical characteristics of a two-wire transmission line depend primarily
on the construction of the line. The two-wire line acts like a long capacitor. The
change of its capacitive reactance is noticeable as the frequency applied to it
is changed. Since the long conductors have a magnetic field about them when electrical
energy is being passed through them, they also exhibit the properties of inductance.
The values of inductance and capacitance presented depend on the various physical
factors that we discussed earlier. For example, the type of line used, the dielectric
in the line, and the length of the line must be considered. The effects of the inductive
and capacitive reactances of the line depend on the frequency applied. Since no
dielectric is perfect, electrons manage to move from one conductor to the other
through the dielectric. Each type of two-wire transmission line also has a conductance
value. This conductance value represents the value of the current flow that may
be expected through the insulation. If the line is uniform (all values equal at
each unit length), then one small section of the line may represent several feet.
This illustration of a two-wire transmission line will be used throughout the discussion
of transmission lines; but, keep in mind that the principles presented apply to
all transmission lines. We will explain the theories using LUMPED CONSTANTS and
Distributed CONSTANTS to further simplify these principles.
LUMPED CONSTANTS
A transmission line has the properties of inductance, capacitance, and resistance
just as the more conventional circuits have. Usually, however, the constants in
conventional circuits are lumped into a single device or component. For example,
a coil of wire has the property of inductance. When a certain amount of inductance
is needed in a circuit, a coil of the proper dimensions is inserted. The inductance
of the circuit is lumped into the one component. Two metal plates separated by a
small space, can be used to supply the required capacitance for a circuit. In such
a case, most of the capacitance of the circuit is lumped into this one component.
Similarly, a fixed resistor can be used to supply a certain value of circuit resistance
as a lumped sum. Ideally, a transmission line would also have its constants of inductance,
capacitance, and resistance lumped together, as shown in figure 3-9. Unfortunately,
this is not the case. Transmission line constants are distributed, as described
below.
3-10
- |
Matter, Energy,
and Direct Current |
- |
Alternating Current and Transformers |
- |
Circuit Protection, Control, and Measurement |
- |
Electrical Conductors, Wiring Techniques,
and Schematic Reading |
- |
Generators and Motors |
- |
Electronic Emission, Tubes, and Power Supplies |
- |
Solid-State Devices and Power Supplies |
- |
Amplifiers |
- |
Wave-Generation and Wave-Shaping Circuits |
- |
Wave Propagation, Transmission Lines, and
Antennas |
- |
Microwave Principles |
- |
Modulation Principles |
- |
Introduction to Number Systems and Logic Circuits |
- |
- Introduction to Microelectronics |
- |
Principles of Synchros, Servos, and Gyros |
- |
Introduction to Test Equipment |
- |
Radio-Frequency Communications Principles |
- |
Radar Principles |
- |
The Technician's Handbook, Master Glossary |
- |
Test Methods and Practices |
- |
Introduction to Digital Computers |
- |
Magnetic Recording |
- |
Introduction to Fiber Optics |
Note: Navy Electricity and Electronics Training
Series (NEETS) content is U.S. Navy property in the public domain. |
|