[Go to TOC] RADAR CROSS SECTION (RCS)
Radar cross section is the measure of a target's ability to reflect radar signals in the direction of the radar receiver, i.e. it
is a measure of the ratio of backscatter power per steradian (unit solid angle) in the direction of the radar (from the target) to the power
density that is intercepted by the target. The RCS of a target can be viewed as a comparison of the strength of the reflected signal
from a target to the reflected signal from a perfectly smooth sphere of cross sectional area of 1 m2 as shown in Figure 1 .
The
conceptual definition of RCS includes the fact that not all of the radiated energy falls on the target. A target's RCS (σ) is most easily visualized
as the product of three factors:
σ = Projected cross section x Reflectivity x Directivity . RCS(σ) is used in Section 4-4 for
an equation representing power reradiated from the target. Reflectivity: The percent of intercepted power reradiated (scattered) by the
target. Directivity: The ratio of the power scattered back in the radar's direction to the power that would have been backscattered had
the scattering been uniform in all directions (i.e. isotropically). Figures 2 and 3 show that RCS does not equal geometric area.
For a sphere, the RCS, F = Br2, where r is the radius of the sphere.
The RCS of a sphere is independent of frequency if operating at
sufficiently high frequencies where λ<<Range, and λ<< radius (r). Experimentally, radar return reflected from a target is compared
to the radar return reflected from a sphere which has a frontal or projected area of one square meter (i.e. diameter of about 44 in). Using
the spherical shape aids in field or laboratory measurements since orientation or positioning of the sphere will not affect radar reflection
intensity measurements as a flat plate would. If calibrated, other sources (cylinder, flat plate, or corner reflector, etc.) could be used for
comparative measurements. To reduce drag during tests, towed spheres of 6", 14" or 22" diameter may be used instead of the larger 44"
sphere, and the reference size is 0.018, 0.099 or 0.245 m2 respectively instead of 1 m2. When smaller sized spheres are
used for tests you may be operating at or near where λ-radius. If the results are then scaled to a 1 m2 reference, there may be some
perturbations due to creeping waves. See the discussion at the end of this section for further details.
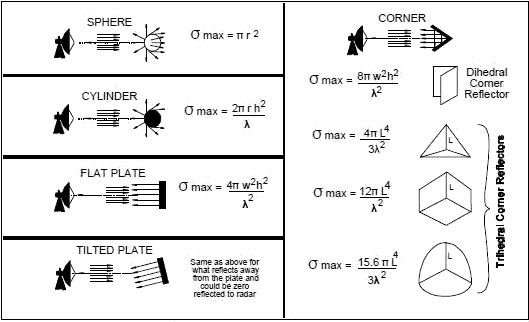
Figure 3. Backscatter From Shapes
In Figure 4, RCS
patterns are shown as objects are rotated about their vertical axes (the arrows indicate the direction of the radar reflections).
The
sphere is essentially the same in all directions. The flat plate has almost no RCS except when aligned directly toward the radar.
The corner reflector has an RCS almost as high as the flat plate but over a wider angle, i.e., over ±60°. The return from a corner reflector
is analogous to that of a flat plate always being perpendicular to your collocated transmitter and receiver. Targets such as ships and
aircraft often have many effective corners. Corners are sometimes used as calibration targets or as decoys, i.e. corner reflectors. An
aircraft target is very complex. It has a great many reflecting elements and shapes. The RCS of real aircraft must be measured. It varies significantly
depending upon the direction of the illuminating radar. Figure 5 shows a typical RCS plot of a jet aircraft. The plot is an azimuth cut
made at zero degrees elevation (on the aircraft horizon). Within the normal radar range of 3-18 GHz, the radar return of an aircraft in a given
direction will vary by a few dB as frequency and polarization vary (the RCS may change by a factor of 2-5). It does not vary as much as the
flat plate.
As shown in Figure 5, the RCS is highest at the aircraft beam due to the large physical area observed by the radar and perpendicular
aspect (increasing reflectivity). The next highest RCS area is the nose/tail area, largely because of reflections off the engines or propellers.
Most self-protection jammers cover a field of view of +/- 60 degrees about the aircraft nose and tail, thus the high RCS on the beam does not
have coverage. Beam coverage is frequently not provided due to inadequate power available to cover all aircraft quadrants, and the side of an
aircraft is theoretically exposed to a threat 30% of the time over the average of all scenarios. Typical radar cross sections are as
follows: Missile 0.5 sq m; Tactical Jet 5 to 100 sq m; Bomber 10 to 1000 sq m; and ships 3,000 to 1,000,000 sq m. RCS can also be expressed
in decibels referenced to a square meter (dBsm) which equals 10 log (RCS in m2). Again, Figure 5 shows that these values can vary dramatically.
The strongest return depicted in the example is 100 m2 in the beam, and the weakest is slightly more than 1 m2 in the
135°/225° positions. These RCS values can be very misleading because other factors may affect the results. For example, phase differences, polarization,
surface imperfections, and material type all greatly affect the results. In the above typical bomber example, the measured RCS may be much greater
than 1000 square meters in certain circumstances (90°, 270°). SIGNIFICANCE OF THE REDUCTION OF RCS If each of
the range or power equations that have an RCS (σ) term is evaluated for the significance of decreasing RCS, Figure 6 results. Therefore, an
RCS reduction can increase aircraft survivability. The equations used in Figure 6 are as follows: Range (radar detection): From the 2-way
range equation in Section 4-4: 
Range (radar burn-through): The crossover equation in Section 4-8 has: 
Power (jammer): Equating the received signal return (Pr) in the two way range equation to the received jammer signal (Pr)
in the one way range equation, the following relationship results: 
Therefore, Pj σ or σ Pj
Note: jammer transmission line loss is combined with the jammer antenna gain to obtain Gt. 
Figure 6. Reduction of RCS Affects Radar Detection, Burn-through, and Jammer
Power
Example of Effects of RCS Reduction - As shown in Figure 6, if the RCS of an aircraft is reduced to 0.75
(75%) of its original value, then (1) the jammer power required to achieve the same effectiveness would be 0.75 (75%) of the original value
(or -1.25 dB). Likewise, (2) If Jammer power is held constant, then burn-through range is 0.87 (87%) of its original value (-1.25 dB), and (3)
the detection range of the radar for the smaller RCS target (jamming not considered) is 0.93 (93%) of its original value (-1.25 dB).
OPTICAL / MIE / RAYLEIGH REGIONS Figure 7 shows the different regions applicable for computing the RCS of
a sphere. The optical region (“far field” counterpart) rules apply when 2Br/λ > 10. In this region, the RCS of a sphere is independent of
frequency. Here, the RCS of a sphere, σ = πr2. The RCS equation breaks down primarily due to creeping waves in the
area where λ-2πr. This area is known as the Mie or resonance region. If we were using a 6" diameter sphere, this
frequency would be 0.6 GHz. (Any frequency ten times higher, or above 6 GHz, would give expected results). The largest positive perturbation
(point A) occurs at exactly 0.6 GHz where the RCS would be 4 times higher than the RCS computed using the optical region formula. Just slightly
above 0.6 GHz a minimum occurs (point B) and the actual RCS would be 0.26 times the value calculated by using the optical region formula. If
we used a one meter diameter sphere, the perturbations would occur at 95 MHz, so any frequency above 950 MHz (-1 GHz) would give predicted results.
CREEPING WAVES The initial RCS assumptions presume that we are operating in the optical region (λ<<Range and
λ<<radius). There is a region where specular reflected (mirrored) waves combine with back scattered creeping waves both constructively
and destructively as shown in Figure 8. Creeping waves are tangential to a smooth surface and follow the "shadow" region of the body. They occur
when the circumference of the sphere - λ and typically add about 1 m2 to the RCS at certain frequencies. 
Figure 7. Radar Cross Section of a Sphere
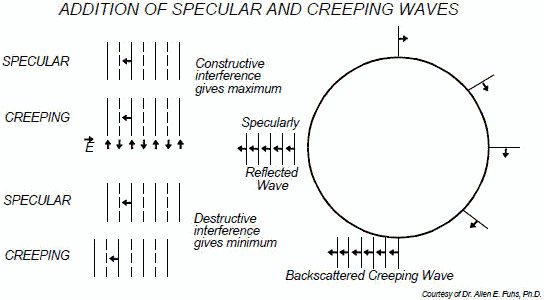
Figure 8. Addition of Specular and Creeping Waves
Table of Contents for Electronics Warfare and Radar Engineering Handbook
Introduction |
Abbreviations | Decibel | Duty
Cycle | Doppler Shift | Radar Horizon / Line
of Sight | Propagation Time / Resolution | Modulation
| Transforms / Wavelets | Antenna Introduction
/ Basics | Polarization | Radiation Patterns |
Frequency / Phase Effects of Antennas |
Antenna Near Field | Radiation Hazards |
Power Density | One-Way Radar Equation / RF Propagation
| Two-Way Radar Equation (Monostatic) |
Alternate Two-Way Radar Equation |
Two-Way Radar Equation (Bistatic) |
Jamming to Signal (J/S) Ratio - Constant Power [Saturated] Jamming
| Support Jamming | Radar Cross Section (RCS) |
Emission Control (EMCON) | RF Atmospheric
Absorption / Ducting | Receiver Sensitivity / Noise |
Receiver Types and Characteristics |
General Radar Display Types |
IFF - Identification - Friend or Foe | Receiver
Tests | Signal Sorting Methods and Direction Finding |
Voltage Standing Wave Ratio (VSWR) / Reflection Coefficient / Return
Loss / Mismatch Loss | Microwave Coaxial Connectors |
Power Dividers/Combiner and Directional Couplers |
Attenuators / Filters / DC Blocks |
Terminations / Dummy Loads | Circulators
and Diplexers | Mixers and Frequency Discriminators |
Detectors | Microwave Measurements |
Microwave Waveguides and Coaxial Cable |
Electro-Optics | Laser Safety |
Mach Number and Airspeed vs. Altitude Mach Number |
EMP/ Aircraft Dimensions | Data Busses | RS-232 Interface
| RS-422 Balanced Voltage Interface | RS-485 Interface |
IEEE-488 Interface Bus (HP-IB/GP-IB) | MIL-STD-1553 &
1773 Data Bus | This HTML version may be printed but not reproduced on websites.
|